1. INTRODUCTION
Phenomena of Pi2 magnetic pulsations (6.7 to 25 mHz - 40 to 150 sec) are associated with the substorm onset. The excitation of Pi2 pulsation is still one of the important subjects in the area of the magnetospheric ultra low frequency waves. The main characteristics of Pi2 pulsations are as follows: (1) they are observed at nightside and dayside region (Sutcliffe & Yumoto 1989, 1991;Ghamry et al. 2011, 2012;Ghamry & Fathy 2016); (2) they are, in general, compressional events (Lee 1998); (3) they are detected in the low-latitude regions (L < 5) on both ground-based and space measurements (Lee 1998). Yeoman & Orr (1989) showed there are a couple of modes for the Pi2 generation mechanisms. The first is the plasmapause surface wave (Sutcliffe 1975) and the second mechanism is the plasmaspheric cavity mode (Allan et al. 1986;Zhu & Kivelson 1989;Fujita & Glassmeier 1995;Lee 1996). Kepko & Kivelson (1999) showed that the mid-latitude/ low-latitude Pi2 pulsations can be driven by fast-mode pulses in the near-Earth magnetotail. Takahashi et al. (2001, 2003) reported the excitation of cavity mode properties in the inner magnetosphere based on satellite observations. Teramoto et al. (2011) used cluster satellites inside the plasmasphere and a satellite in the plasmatrough to provide additional evidence of plasmaspheric virtual resonance based on observations of the Pi2 pulsations using two satellites at different regions in the inner magnetosphere. Ghamry et al. (2015) made an observation for Pi2 pulsations using Van Allen probes (VAP-A & VAP-B). According to the study show, the Pi2 pulsations observed outside the plasmasphere support the plasmaspheric virtual resonance model.
Although Pi2 can be associated with substorms, Pi2 can also occur with periods and waveforms similar to classical Pi2 pulsations without the occurrence of substorms. Sutcliffe (1998) reported Pi2 pulsation that occurred during quiet conditions on 10 March 1997. Lyons et al. (1999) investigated quiet-time Pi2 pulsations in detail. Kwon et al. (2013) statistically examined the quiet-time Pi2 events observed on a ground station in South Korea, in the year 2008. Ghamry (2015) reported a morningside Pi2 pulsation that occurred in the absence of substorm in very quiet geomagnetic conditions (Kp = 0). Kwon et al. (2015) studied the plasmapause location in the period of quiet geomagnetic conditions using the electron density from the time history of events and macroscale interactions during substorms (THEMIS). Until now, however, there is no statistical study of the Pi2 pulsations using satellite observations during extremely quiet conditions when Kp = 0 to the best of the author’s knowledge. Also, no attention has been paid to Pi2 pulsation in the space fluxgate magnetometers near perigee. In this study, a statistical investigation on the Pi2 pulsations observed under the very quiet geomagnetic conditions (Kp = 0) using Van Allen probe satellites for the period from October 2012 to December 2014. All of Pi2 events detected did not show sudden decreases in auroral electrojet index AL. Also, Pi2 pulsation in the space magnetometers near perigee was studied.
The organization of the paper is as follows. Section 2 de-scribes the data sets and Section 3 presents an example of Pi2 pulsations when Kp = 0. The statistical analysis is described in Section 4. In Section 5, twenty two Pi2 pulsations where L < 4 are presented. In Section 6, we discuss the results and Section 7 gives the conclusions.
2. DATA SETS
By using Kakioka (KAK, L = 1.23) magnetic field records in Japan as a reference signal to identify Pi2 pulsations, observation was made during extremely quiet geomagnetic conditions (Kp = 0), in the nighttime (1800–0600 local time; LT = UT + 9 hours). Then the Pi2 events occurred in the nighttime (1800– 0600 magnetic local time) at Van Allen probes (VAP-A & VAP-B) were visually examined to exclude waves excited by solar wind disturbances. The twin Van Allen probe (VAP) spacecraft, launched on 30 August 2012, makes a near-equatorial orbit with apogee (L ~ 6 RE) and perigee (L ~ 700 km) (Mauk et al. 2013).
VAP consists of two spacecrafts (VAP-A and VAP-B) and both have identical triaxial fluxgate magnetometers capable of measuring 64 vectors per second (Kletzing et al. 2013). Electric and magnetic field instrument suite and integrated science (EMFISIS) (Kletzing et al. 2013) was used to get magnetic field data. We rotated the EMFISIS magnetic field data of the geocentric solar magnetospheric (GSM) coordinate system into a mean field aligned (MFA) coordinate system. This decomposition allows the dominant magnetic field wave polarization to be determined as toroidal (azimuthal), poloidal (radial), or compressional (parallel). In this system, Ez is in the direction of the mean magnetic field, Ey (eastward) is parallel to Ez × r, where r is the position vector of the satellite relative to the center of the Earth; and Ex (radial) is given by Ex = Ey × Ez.
The electric field data were provided in the modified geocentric solar ecliptic (MGSE) coordinates in which Ey and Ez are in the satellite spin plane, with Ey pointing duskward (nearly the same as Ygse), and Ez pointing nearly along the positive normal to the ecliptic. The y and z components in MGSE were measured directly by electric field and waves (EFW) instrument (Wygant et al. 2013). The parallel component, δBz defined by Bz (1 sec) minus Bz (5 min average) is the high-pass filtered compressional component. The data has different resolution for the components. It is 1 sec for the VAP magnetic field, 11 sec for the VAP electric field and 1 sec for the KAK magnetic field. For frequency domain analysis, 12 sec averages for all components are used.
3. AN EXAMPLE OF Pi2 PULSATIONS WHEN Kp = 0
The provisional auroral electrojet index AL & AE and the horizontal component of the magnetic field H (northward), from KAK, as shown in Figs. 1(a) and 1(b), respectively, from 13:05 to 13:40 UT on 31 December 2012. AE index ranges from -20 nT at 1308 UT to -31 nT at 1317 UT. Although AL is negative, its magnitude is small (< 34 nT), and decreases during a typical substorm. Therefore, a substorm current wedge was coherence and cross phase. We compute the power spectral density, coherence, and cross phase for the 35 min interval from 1305 UT to 1340 UT using Fourier transform with five point smoothing in the frequency domain. The cross phase is calculated for frequencies at which the coherence is greater than 0.7. The spectral parameters are plotted in Figs. 3(a)-3(c). The δBz data from VAP-A show a power spectrum similar to the KAK spectrum in the frequency band from 3 to 12 mHz. In this band, the coherences of KAK-VAP-A δBz and KAK-VAP-A δBx are higher than 0.7. The Fig. 3(c) show a mixture of the fundamental (~4 mHz) and second harmonic (~8 mHz). The cross phase between KAK-VAP-A δBz and KAK-VAP-A δBx are about 180° and about 270°, respectively.
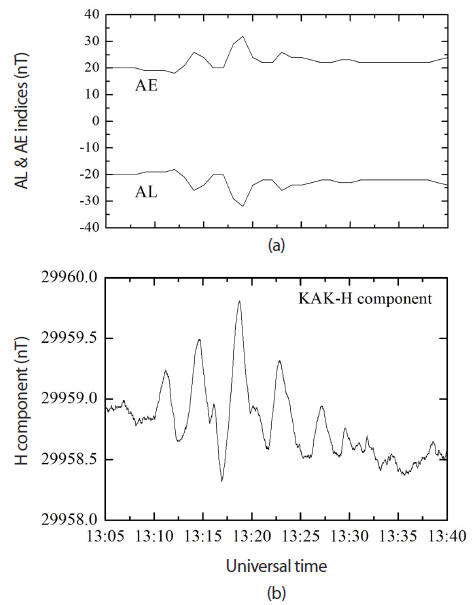
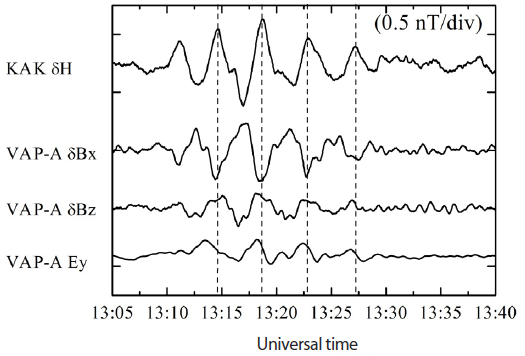
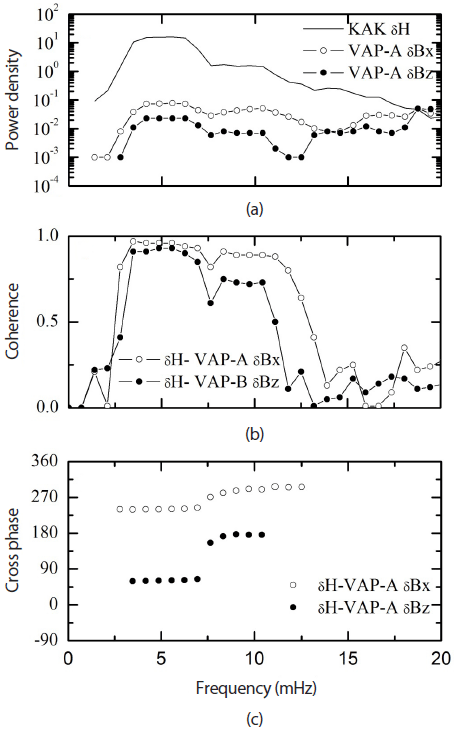
4. STATISTICAL RESULTS
A statistical analysis of the ninety six Pi2 pulsations iden-tified by VAP-A and VAP-B was performed. Fig. 4 illustrates the location of the VAP in the SM (solar magnetic) coordinates for all Pi2 events. Fig. 5 shows the ninety six events distribution not formed during the Pi2 event (Clauer & McPherron 1974). As per this observation, it is suggested that the Pi2 pulsation presented in Fig. 1 occurred in the absence of a substorm.
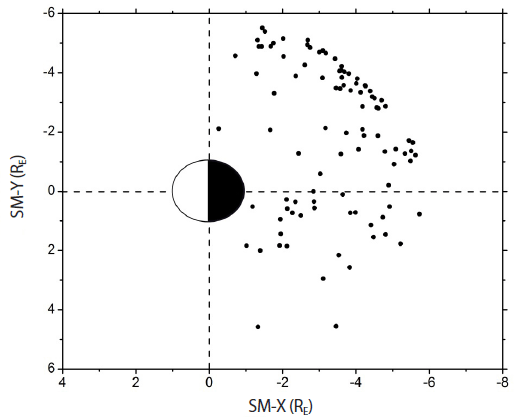
Space observations are important to examine the properties of magnetic and electric field perturbations in space which correspond to Pi2 pulsations on the ground. We have compared the low latitude horizontal H component on the ground and the poloidal components in space which are characterized by the azimuthal oscillation of the electric field (Ey), the radial (δBx) and compressional (δBz) oscillations of the magnetic field in Fig. 2. To identify the phase delay, the vertical dashed lines in Fig. 2 is drawn through the peaks of δH component.
During the time of interest (UT = 13:05-13:40), the VAP-A was in south of the magnetic equator with a magnetic latitude between -9.28 and -9.72, and it moved outward from L = 4.0 to L = 4.8 post-midnight while KAK was in premidnight. Both the VAP-A and KAK were found on the night side separated by a small local time (~ 3 hours).
As shown in Fig. 2, VAP-A observed a compressional (δBz) component with period and waveform similar to the H com-ponent at KAK. This gives us an indication that the oscillations at KAK and VAP-A are excited by a similar source. The radial magnetic field component, δBx, at VAP-A oscillates out of phase with δBz. The vertical dashed lines, in Fig. 2, indicate that the δBz peaks precede the Ey peaks by a quarter of the wave period, which is equivalent to a phase delay of ~90°. These phase relationships among the poloidal components (δBx, δBz, and Ey) are considered as an indication to the fundamental mode (Takahashi et al. 2001, 2003). One can note that δBx is larger than δBz by a factor of ~2. It is believed that VAP-A was near the node in the fundamental mode δBz perturbation (Takahashi et al. 1995).
The frequency analysis was used in this study because it gives additional details on the frequency dependence of the according to magnetic local time (MLT). In Fig. 5, the Pi2 events are distributed from 01:00 to 05:00 MLT, and relatively small number of pulsations are observed from 19:00 to 00:00 LT.
Fig. 6 shows the Pi2 frequency distribution based on magnetic local time. We found seventy five events have power spectra ranging from 5 to12 mHz, which differ from the regular Pi2 band range from 6.7 to 25 mHz (Troitskaya 1967). The Pi2 power has the highest value at 20-23 MLT, and there was an asymmetry phenomenon of Pi2 power around the midnight. We examined the coherence between KAK H and the poloidal components at VAP. We found seventy five pulsations with high coherence between VAP and H components at KAK station. In this study, the meaning of high coherence is that the H component at KAK and one of the poloidal components at VAP satellite have spectral peaks at the same frequency, and at this frequency, the coherence is higher than 0.7.
5. Pi2 PULSATIONS WHERE L < 4
In this section, we examined twenty two clear Pi2 pulsations, detected by the fluxgate magnetometer of the VAP-A and VAP-B near the perigee (L < 4) for the same period (2012-2014). KAK is chosen as a reference station to determine the relative amplitude and phase of the Pi2 observed by VAP-A and VAP-B. Table 1 displays a list of twenty two Pi2: event number, date, start time, end time, VAP, L-value, and MLT of VAP. We have made four clear case studies of Pi2 where L < 4 (at the onset time of Pi2). In each column, in Figs. 7(a)-7(d), top panel shows the time series plot of the δH component at KAK and the δBz components of VAP, second panel shows the power spectra, the third panel shows the coherence, and the cross phase is plotted in the fourth panel. We removed a second-order polynomial of δBz components fitted to the original time series using least-squares method. During 1815-1835 UT on 7 October 2012 the VAP-A was in north of the magnetic equator with a magnetic latitude 3.3, and it moved outward from L = 1.3 to L = 2 from premidnight to postmidnight (MLT = 2225-0125) while KAK was in postmidnight (MLT = 0315-0335). On 27 February 2013, during 2045-2100 UT, the VAP-A was in south of the magnetic equator with a magnetic latitude between -5.2 and -6.7, and it moved outward from L = 2.2 to L = 2.4 premidnight (MLT= 2005-2035) and KAK was in dawn (MLT= 0545-0600). During 1320-1340 UT on 1 January 2013 the VAP-A was in south of the magnetic equator with a magnetic latitude around -14, and it moved outward from L = 3.2 to L = 3.6 midnight (MLT~ 0000) and KAK was in premidnight (MLT= 2120-2140). On 19 April 2013, during 1540-1605 UT, the VAP-A was in north of the magnetic equator with a magnetic latitude around 2, and it moved inward from L = 3.9 to L = 3.4 postmidnight (MLT~ 0200) while KAK was in midnight (MLT= 0040-0105).
![]() |
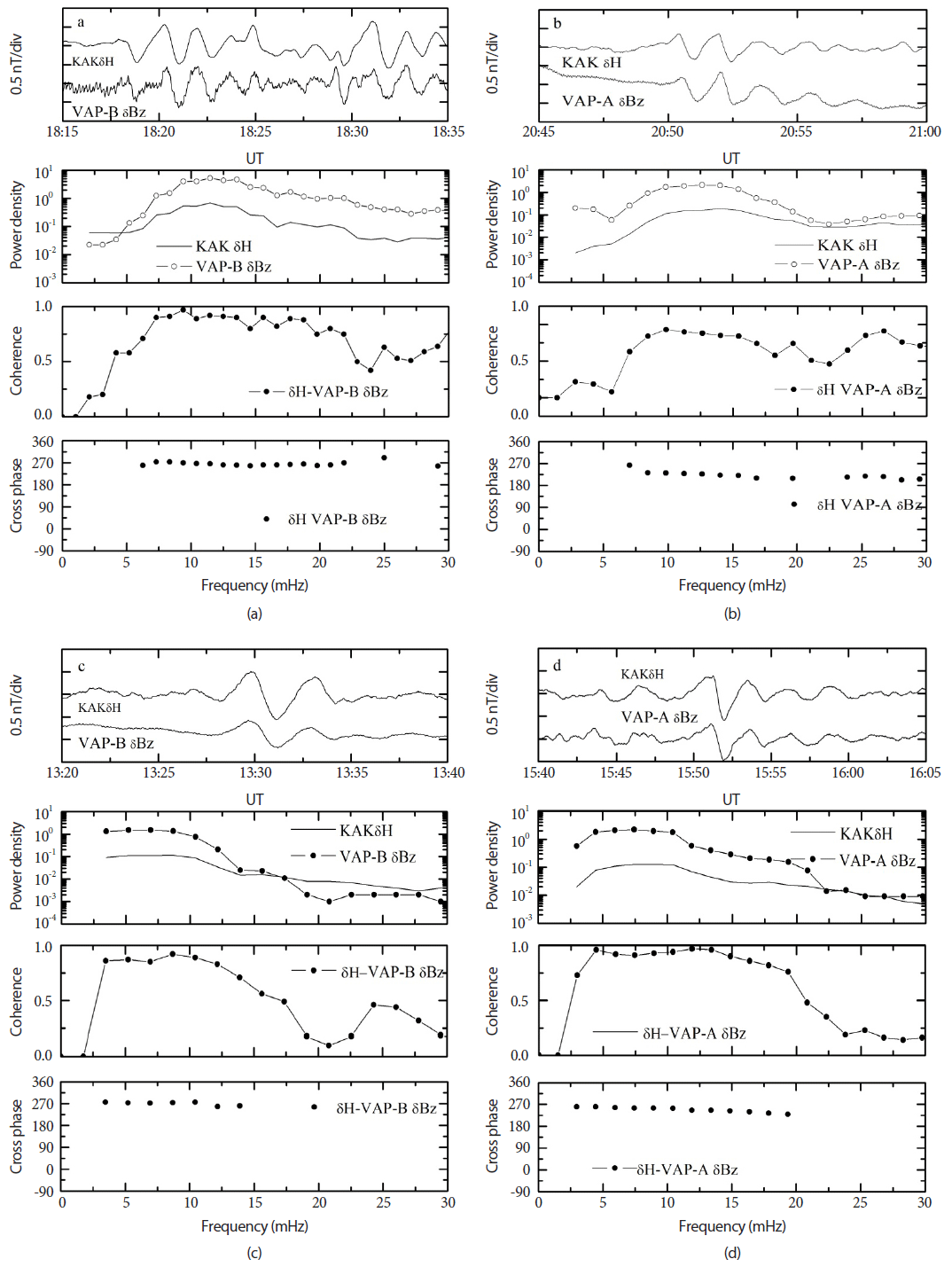
6. DISCUSSION
Satellite observation of magnetic pulsation, Pi2, at sub-storm onset at L > 6.6 are well documented (Arthur & McPherron 1977). One of the objectives of the present study is to statistically examine Pi2 pulsations observed during the most quiet geomagnetic conditions (Kp = 0) where L < 6 at VAP-A and VAP-B satellites. While Kp = 0, we could consider the magnetosphere to be in a near ground state. Most of Pi2 pulsations were detected without sudden decreases in AL index. We found that seventy five events had high coherence between VAP-Bz and H components at KAK station. We also, found that 77 % of events were observed in the range between 5 and 12 mHz, which is smaller than the typical Pi2 power range of 6.7-25 mHz. It is known that the radially trapped fast mode waves in the plasmasphere are one of the source mechanism of low-latitude Pi2 pulsations. The evidence for the plasmaspheric resonance mode has been provided from satellite observations in the inner magnetosphere (e.g., Takahashi et al. 1995). The size of the plasmasphere is considered as one of the factors that controls the plasmaspheric resonance frequency. Takahashi et al. (2003) showed that Pi2 frequency decreases as the plasmapause distance determined using electron density data from a spacecraft, increases and that Pi2 frequencies are in the range of ~4-8 mHz (periods from 125 to 250 sec) when the plasmapause is above L = 6. Chappell et al. (1970) suggested a relationship between the Kp index and the plasmapause distance. From this relationship, it can be expected that the plasmapause is located somewhere above L = 6 for Kp = 1. There is another feature of low-latitude Pi2 pulsations that it depends on the local time as shown in the Pi2 pulsation power distribution. As shown in Fig. 6, the Pi2 power data based on local time indicate that four Pi2 power has the maximum value at 20-23 MLT, and the asymmetry of Pi2 power occurs around the midnight. This result is similar to the result of Takahashi & Liou (2004). The Pi2 pulsation power depending on local time is higher in the pre-midnight portion
(Takahashi & Liou 2004). This is because the highest point of auroral power is around 21 LT and the source region of Pi2 pulsations is located near the eastern head of auroral surge. However, the exact cause is not yet identified. In this study, only four Pi2 pulsation powers were higher in the pre-midnight portion than in the post-midnight portion, six Pi2 pulsation power spectra showed the higher value in post-midnight portion, and the highest Pi2 pulsation power spectrum was observed between 20 and 23 MLT.
Another target of this study is to search the space magnetic data for clean Pi2 pulsations where L < 4. In space, the electric field data are sensitive to Pi2 pulsations down to L ~ 2 but the magnetic field data are very noisy to investigate Pi2 pulsations at L < 4 (Takahashi et al. 2003). That's why the Pi2 observation from magnetic data near satellite perigee did not attract any attention. In this study, fortunately, we could obtain twenty two clean Pi2 pulsation by the fluxgate magnetometer of the VAP-A and VAP-B near perigee (L < 4). The compressional components showed Pi2 oscillations on L shells as low as ~1. This enabled us to see the relationship among the compressional components at VAP and H com-ponent at KAK for events observed at various L distances. We found that the compressional components at VAP of all events have high coherence with H component at KAK with nearly identical waveforms. We presented four example of Pi2 oscillations on different L shells (L < 4). The four examples show similar spectral characteristics (Figs. 7(a)-7(d)). All the δBz data give a power spectrum nearly identical to the δH spectrum in the frequency band range from 5 to 20 mHz. The cross phase between KAK δH and VAP-δBz is about 270° in all cases. It should be noted that this study, related to Pi2 near perigee, is to show the possibility to detect Pi2 events from space magnetometer where L < 4 with high coherence of compressional components with ground H component. However, twenty two events are not enough to find out the mode structure of these events. We need to extend our study to cover more data sets focusing on events near perigee.
Integrated interpretation of magnetic field data from the magnetosphere, ionosphere and ground can lead to better understanding of Pi2 wave occurrence and propagation. Thus, other studies of Pi2 events recorded simultaneously in several locations of the geospace are necessary and a mission like Swarm in the topside ionosphere, is expected to help with resolving a lot of Pi2 issues. Presently, a study is under progress on the relationship between Pi2 occurrence in the low-latitude and the magnetic field measured by VAP and Swarm satellites.
7. CONCLUSION
A statistical analysis of the Pi2 pulsations has been made using the VAP-A and VAP-B satellites when Kp = 0 from October 2012 to December 2014. During this period, ninety six events were detected. In this study, seventy five events had high coherence between VAP-Bz and H components at the KAK station. We found that 77 % of the events had a power spectrum between 5 and 12 mHz, which differs from the regular Pi2 band range from 6.7 to 25 mHz. In addition, this study demonstrates that it is possible to observe Pi2 pulsations from space fluxgate magnetometers near perigee. Twenty two clean Pi2 pulsations were detected and listed using the Van Allen probe fluxgate magnetometer where L < 4. Four examples of Pi2 oscillations on different L shells near perigee were presented. The four examples show similar spectral characteristics.