1 NTRODUCTION
While Higgs boson enables the Standard Model (SM) to be established (ATLAS Collaboration 2012; CMS Collaboration 2012), it has been still noted to be imperfect since the gravitation force was not included from the equation for the four forces working in nature. At the current scale of energy, the SM has been shown to be accurate in explaining the phenomena. However, it will be improper at the high energy scale. The universe is well known to be consists of 70 % dark energy, 26 % dark matter and 4 % SM particles (Cho 2016a).
Therefore, a research on dark matter provides the evidence of physics beyond Standard Model. Its mass is not well understood. It is thought that weak scale, masses, and coupling requires many corrections. In models, there are good candidates which are designed to understand the weak scale. Significant efforts on experiments are also required such as Large Hadron Collider (LHC) experiments. However, hitherto, LHC experiments do not show much new physics. Dark matter is perhaps not linked with the weak scale or it could be part of a complicated sector corresponding to that of the visible sector. Therefore, dark matter should be investigated comprehensively.
The necessary conditions of dark matter known hitherto are that any type of light is not emitted since it escapes. It almost does not interacts with any matter. And it is cold since its velocity is almost zero in the early universe (Cho 2016b). There are three experimental methods: direct detection, indirect detection and accelerator detection (Cho 2017). Table 1 shows the experimental types for the accelerator detection (Alexander et al. 2016).
Among the experimental types of dark matter using an accelerator, we review the studies on dark matter with the e+e− collider. This experiment yields the following strong points. It is an intensity frontier. As is a fixed center of mass (CM) energy, it provides a good constraint condition. It is also sensitive to light dark matter mass between a few MeV/c2 and 5 GeV/c2. It is relatively free from unitarity or the validity of the effective field theory (EFT). It gives a clean signal and low background compared to the hadron collider (Cho 2017). The results are possible from both prompt decay and displacement decay. Fig. 1 shows the location where the e+e− collider data are produced. Table 2 shows the e+e− collider experiments.
2 DARK MATTER WITH e+e− COLLIDER
As each experiment and each theory uses its own naming, we categorized the same meanings in Table 3.
Fig. 2 shows the scheme of dark sector is modified from the SM. The dark sector scheme starts from the SM system towards the dark sector.
For the connection between dark sector and the SM, our photon (γ), which is visible, is in the SM while the dark photon (A') is invisible beyond Standard Model where ε2 is the mixing strength. Fig. 3 shows the typical channels for the dark photon generation and the Higgsstrahlung channel. Fig. 4
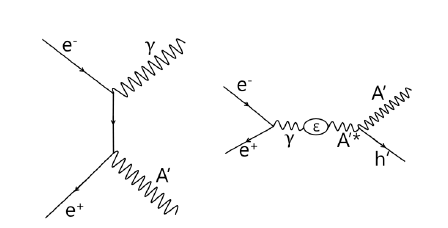
Herein, we name the dark sector type as the following four types:
-
Type 1. Dark photon that decays to dark matter
-
Type 2. Dark photon that decays to the SM particles
-
Type 3. Higgsstrahlung
-
Type 4. Dark photon that couples only to heavy leptons.
Herein, each type is described.
Type 1. Dark Photon that Decays to Dark Matter case, nothing is left in the detector.
Fig. 5 shows the plane of MA' vs. Mχ with different characteristics of the single-photon and missing ET signals. A' decays to the dark matter on-shell or off-shell with different gamma spectrum. The results are from the radiative production in an collision. In the final state, there exists only single-photon with the energy, Here, s is the square of CM energy at the e+e− collider and is dark photon mass (Essig et al. 2013). This requires a high single-photon trigger. However, it is not available in the Belle experiment, while the BaBar experiment had been implemented with a single-photon trigger (BABAR Collaboration 2008). It will be implemented in the Belle II experiment.
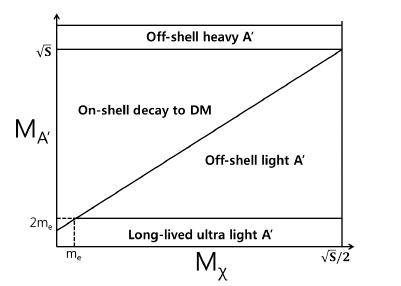
As shown in Fig. 6, dark photon A' can decay directly to the SM (Batell et al. 2009). Fig .7 shows branching ratios of each decay channel (Batell et al. 2009).
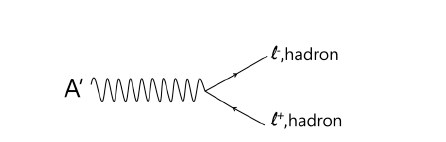
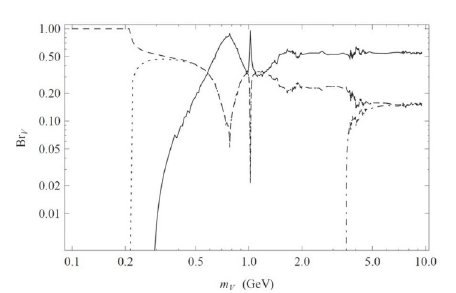
The cross section of dark photon is inversely proportional to the square of the CM energy (See the left Feynman diagram of Fig. 3). Therefore, it requires low energy (1–10 GeV) with a high-intensity collider (BaBar, Belle and KLOE). A broad direction search is required and underway.
Fig. 8 shows the Feynman diagram of the Higgsstrahlung. Here, h' decays depending on Mh' and MA'. We can construct a two-dimensional plot for Mh' and MA' to measure the coupling constant between the dark photon to the dark Higgs, αD. As shown in Fig. 9, three cases exist:
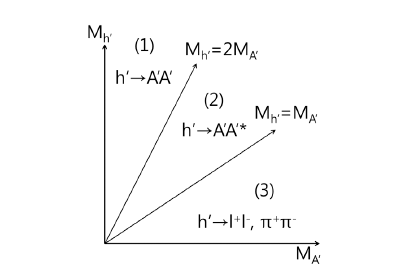
Case (1): Mh' > 2 MA' : h' → A'A', very low background
Case (2): MA' < Mh' < 2 MA' : h' → A'A'*
Case (3): Mh' < MA' : h' is long lived and h' → l+ l−, π+ π−
This is rather similar to that of type 2. If the SM fields are under a dark force and charged, the lepton universality may be broken. A' may decay only to heavy leptons (Shuve & Yavin 2014). The theory can explain the muon anomalous magnetic moment. Α' → υν̅ if A' couples. Fig. 10 shows the Feynman diagram of e+e− → μ+μ−A' and A' → μ+μ− decay channels.
3 RESULTS
We have reviewed the KLOE experiment, BES III experiment, BaBar experiment, Belle experiment, and Belle II experiment. Table 4 shows the search for dark mattes types for each experiment, and the summary of the final states depending on the dark matter types.
The KLOE experiment is located in Italy. The CM energy is 1,019.4 MeV, which is the resonance state of ϕ (e+e− → ϕ). The physics programs are kaon physics, spectroscopy, hadron cross section, γγ physics, and dark sector.
The first search is the dark photon (ηe+e−), as follows (KLOE-2 Collaboration 2016a):
Fig. 11 shows the Feynman diagram with the final state of ηe+e−.
The second search is the dark photon (e+e−γ & μ+μ−γ). Fig. 12 shows the final state Feynman diagram of e+e−γ or μ+μ−γ (KLOE-2 Collaboration 2016a).
KLOE investigates in the dark photon mass range below 1 GeV from the motivation of light gauge boson. The final state is π+π-γ or μ+μ-γ. No invisible decay is assumed due to MA' < Mχ. The evidence for a signal does not exist. KLOE set a limit at 90 % confidence level (CL) on ε2 in the MA' range of 527- 987 MeV, which is more constraint than the previous ones beyond 700 MeV (KLOE-2 Collaboration 2016b).
As shown in Fig. 13, the final state is μ+μ− and missing ET (h'). This is case (3) Higgssrahlung described in section 2.1.3. The h' long lived and invisible. The evidence of signal does not exist. KLOE set a limit on 90 % CL to its parameters in the range 2Mμ < MA' < 1,000 MeV, Mh' < MA' (KLOE-2 Collaboration 2015).
The BES III experiment is located in Beijing, China. It is a charm factory for τ-charm physics. The physics program are charmonium-like physics, light hadron spectroscopy, charm physics, and tau physics. GeV is the collision energy (BES III Collaboration 2017).
Using 2.93 fb−1 dataset, BES III searches for a dark photon. BES III examines the initial state radiation reactions as follows:
In this search, the invariant mass distribution of lepton pairs appears an enhancement as dark photon. The evidence of signal does not exist.in the mass range of (1.5 - 3.4) GeV/c2. BES III set a limit on 90 % on the mixing strength, ε2 (BES III Collaboration 2017).
The BaBar experiment produced ~533 fb−1 dataset around the Υ(4S) in 1999–2008. The following data samples were collected: ~470 × 106 Υ(4S), ~120 × 106 Υ(3S) and ~100 × 106 Υ(2S) (BABAR Collaboration 2008).
The BaBar experiment has one photon + with missing ET trigger. Therefore, this experiment measures the decay channel of
The first search was Υ(3S) →γA' and A' → invisible (BABAR Collaboration 2008). Through the next-to-minimal supersymmetric SM (NNSSM), such an object appears. BaBar investigate the events of single-photon with large missing ET by tagging the two-body decay of Υ(3S). The evidence of signal does not exist for such processes in 122 × 106 Υ(3S) events. BaBar set a upper limit on 90 % CL on the branching ratio of B(Υ(3S) → γ A') × B(A' → invisible) at (0.7−31) × 106 in the dark photon mass range MA' ≤ 7.8 GeV (BABAR Collaboration 2008).
The second search was Υ(2S) → π+π− Υ(1S), Υ(1S)→γA0, where A0 decays invisibly. A0 is invisible. It is either a light Higgs boson with zero spin, or a dark matter pair with zero spin. BaBar investigate the events of single-photon with large missing ET by Y(1S) tagging (BABAR Collaboration 2011). The evidence of signal for such processes does not exist in the range MA0 ≤ 9.2 GeV/c2 and Mχ ≤ 4.5 GeV/c2 using 98 × 106 Υ(2S) events. BaBar set an upper limit on the model which has light dark matter. The results are:
at 90 % CL upper limits (BABAR Collaboration 2011).
BaBar searches for e+e− → Υ(2S, 3S), Υ(2S, 3S)→ π+π− Υ(1S), Υ(1S)→γA0 and A0 → μ+μ− (BABAR Collaboration 2013). BaBar selects Y(1S) events by tagging the pion pair. The evidence of signal does not exist for the A0 production examining 116.8 × 106 Υ(3S) events and 92.8 × 106 Υ(2S) events. BaBar set an upper limit on 90 % CL on the product branching ratio as follows:
Using 516 fb−1 dataset, BaBar experiment searches for the dark Higgs boson and set the upper limits (BABAR Collaboration 2012). This is case (1) Higgsstrahlung in Section 2.1.2. The experiment set a limit on 90 % CL on the crosssection on e+e− → hA' and h' → A'A depending on h' and A' masses. The experiment uses the conservative approach with no background subtraction (BABAR Collaboration 2012).
The channel is e+e− → μ+μ− Z' and Z' → μ+μ−. The Z' lepton coupled the dark photon mode. Using 514 fb−1 dataset, BaBar searches for the Z' which couples only to heavy leptons. The evidence of signal does not exist for the Z' mass range of (0.212 − 10) GeV. BaBar set the lower limit on the coupling parameter g' as 7 × 10−4, which improves the bounds compared to neutrino experiments (BABAR Collaboration 2016).
The Belle experiment produced ~1 ab−1 data taken at KEK, Japan in 1999–2008 (BABAR Collaboration 2016).
Type 1 requires a high single-photon trigger. This is not available in the Belle experiment while BaBar was implemented with a single-photon trigger (BABAR Collaboration 2008).
Dark photon decays to the SM particles such as e+e−, μ+μ− and π+π− (Kacurova 2009). Here, the dark photon mass range is between (0.1−10 ) GeV/c2. This analysis includes the dark photon decays from prompt or a displaced vertex. Fig. 14 shows the decay channel with the displaced vertex. This is also called the “long-lived gauge boson” instead of dark photon. This work is underway. Belle also investigate a dark vector gauge boson which decays to π+π− by tagging D*0 decays (Belle Collaboration 2016). The evidence of signal does not exist. Belle set a mass-dependent limit on the baryonic fine structure constant of 10−3 − 10−2 in the dark photon mass range of 290-520 MeV/c2 (Belle Collaboration 2016).
The Belle experiment searches for the Higgsstrahlung case (1) described in section 2.1.3. It has very low background by prompting h' and A'. The Belle measures exclusively three pairs of charged track with the same invariant mass and total energy of event, inclusively two pairs of charged track with the same invariant mass, and the third A' from the four momenta of the e+e− collider beam constraint system. In conclusion, Belle used 10 exclusive channels: 3(l+l−), 2(l+l−) (π+π−), 2(π+π−) (l+l−), 3(π+π−) with l = e, μ, and three inclusive channels: 2(l+l−)X with X dark photon (missing mass). The evidence of signal does not exist. Belle set a limit on 90 % CL on the mixing strength (Belle Collaboration 2015).
Belle II experiment has started using e+e− collision at ∼10.3 GeV at KEK, Japan in 2018. Belle II will obtain a full luminosity of 50 ab-1. It has sensitivity to visible (γl+l−) and invisible (single-photon) modes. Belle II will also search for rich final states such as e+e− → h'A' → A'A'A'. Due to the advantage of Belle II no-projective-cracks design, Belle II develops trigger strategies for monophoton modes. The range of sensitivity in dark photon mass is 20 MeV/c2 – 10 GeV/c2 (Essig et al. 2013; Alexander et al. 2016).
For the one photon + with missing ET for the channel, a single-photon trigger is required. Belle II develops it.
The resolution of invariant mass of μ+μ− at the Belle II experiment is much better than that of Belle experiment. Therefore, Belle II has much better efficiency for these channels (Alexander et al. 2016).
The Belle II experiment also proposed to search axionlike particles (ALP), as shown in Fig. 15. They are pseudoscalars and couple to bosons. Unlike QCD axions, ALPs have no relation between mass and coupling, assuming all axions decay into two photons a → γγ. As shown in Fig. 16, four regions exist on the plane of gAγγ−mA: the invisible, displaced, merged, and resolved. For the invisible region, the ALP decays outside of the detector or into the invisible particles: the single-photon final state. For the displaced region, the searches for invisible and visible APL decays into this region. For the merged region, two of the photons overlap or merge. For the resolved region, three resolved high energetic photons (Mimasu & Sanz 2014).
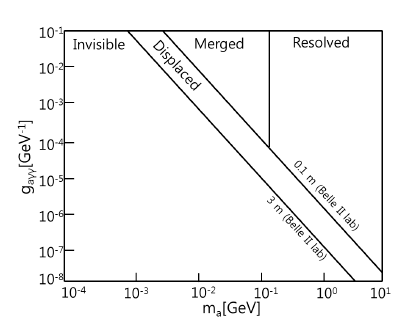
4 SUMMARY
The e+e− collider experiment (KLOE, BESII, BaBar, Belle and Belle II) contributes to searching for light dark matter particles. Table 5 shows the summary of the search for dark sector at e+e− experiments. Fig. 17 shows the plane of mixing strength, ε vs. dark photon mass at the limit of 90 % CL. A signal for dark matter or dark photon has yet to be found. Belle II will search for dark matter with the single-photon trigger. Future searches are set to constrain large energy ranges and kinematic mixing parameter values in dark matter researches.
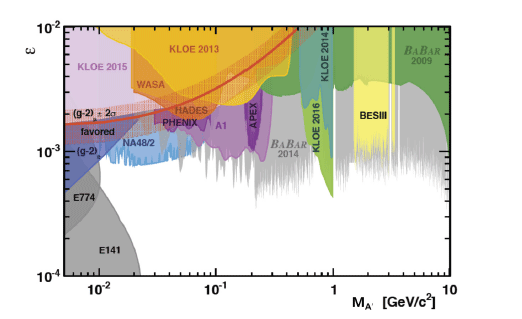