1 INTRODUCTION
Astrobiology is the study and the effort of mankind to find life in other worlds. Since Earth is the only known place to have life, various standards in finding life or habitable places are constructed through observations on Earth. An example is the Viking biological experiment, which was conducted on Mars in 1976. The main goal of the experiment was to find the product of glucose metabolism, the most common life metabolism on Earth. Radioactive carbon isotope-glucose and carbon dioxide was used to detect signs of catabolic reactions (glucose metabolism) or anabolic reactions (photosynthesis) (Klein et al. 1972). Irrespective of its biologically null result, this first attempt to find life on other planet triggered the start of systemized astrobiology study. One of the ultimate goals of remote sensing investigations on the chemical composition (Kim et al. 2018) and surface morphology (Hong et al. 2014, 2015; Jung et al. 2014, 2016) of the planets and their satellites is looking for habitable places of past or current life forms.
In the perspective of the astronomical scale, the current knowledge of life is limited because of the restriction of life samples, which are only collected on Earth. Further-more, with the discoveries of new species, what are considered the features of life are constantly changing. For instance, arsenate, which was considered a toxic material to all life is a substitute of phosphorus for GFAJ-1, a type of bacteria (Wolfe-Simons et al. 2011). Also NA1, a type of archaea, was found to use a unique metabolism that generates their energy by using formate instead of the common glucose metabolism (Kim et al. 2010).
Astrobiology is the study and the effort of mankind to find life in other worlds. To seek for a life in other celestial bodies, designing life-detecting experiments is essential. Since the universal features of life are unclear, broad biological experiment topics are required to search for bio-signatures. One of the newest topics is the virus. So far, biologists define life, considering six specific features (cells, metabolism, actionreaction, development and growth, reproduction and heredity, adaption and evolution). Applying these standards, there is controversy as to the classification of the virus because it does not fulfill the biological standards of life features by itself, requiring a living host. However, tracking its signatures can play a critical role in detection of life and the evolution of the terrestrial biological environment. The signature of viruses can give various information about the habitable conditions because of their unique survival moethod, parasiting.
The detecting technique of active viruses is available. However, since there is no certainty as to the existence of active viruses on other worlds, an experiment that seeks for a signature of the presence of past presence viruses (virus fossils) is needed. Unfortunately, there are no technologies that detect fossils of physical viruses. Therefore, there is as of yet no evidence of existence of the morphological virus fossils. Physically, well preserved virus fossils would significantly contribute to the reproduction of the past history of celestial habitability and to the information of the corresponding environmental conditions. There are methods to track down the genetic information of past viruses, but morphological virus fossils have never been found. One form that could possibly act as a virus fossil was artificially made. In a silicaabundant environment (artificially manipulated), viruses formed fossil-like structures (Laidler & Stedman 2010). Not only was the silicification of viruses reversible, it could also protect the virus from viral-hostile conditions (Laidler et al. 2013). Fig. 1 shows a brief summary of virus silicification. The stability of silicified viruses must be studied in the near future, as only its effective heat and dehydration stability has been studied so far (Wang et al. 2015). The endurance of silicified viruses in dehydrated environments and heat implies the possibility of long distance migration of viruses, explaining identical virus infection across the desert in the past. Although there are many experiments left to test the stability of silicified viruses, considering the nature of this feature, it is not a huge leap to imagine the interplanetary propagation of viruses.
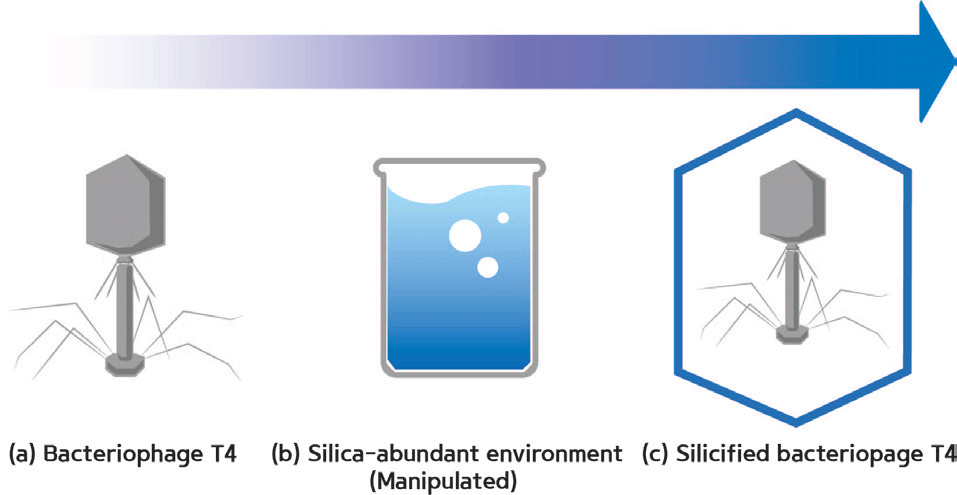
Not only is the series of life-detecting experiments based on Earth standards, but also are the observational targets of habitable celestial bodies based on terrestrial environmental elements. One of the most important elements of these standards is the presence of liquid water. This standard highly suggests Jovian satellites to be habitable because of spectroscopic data showing meaningful amounts of water content, especially in Europa (thick icy shell). Although there are ongoing discussions on the existence of liquid water inside the thick icy shell of Europa, a series of recent studies directly showing transient water jets on the surface of Europa implies high possibility for the existence of liquid water inside the shell (Roth et al. 2014; Sparks et al. 2016).
The next mission to Europa (the Clipper) should conduct detailed data collection of the atmosphere and internal geological data of Europa to prepare the lander mission, which will be the most significant mission in astrobiology history. Atmospheric environmental data should provide a proper landing site for subsequent Europa lander missions, and internal geological data will give the specific energy sources of heat that are expected to keep Europa’s ocean in a liquid state. Furthermore, the mission is also expected to provide data, which will help determine compositions of organic molecules inside the oceans of Europa.
The objective of this study is to propose a conceptual experiment for life-detecting in a future Europa landing mission. The idea can cover a broader range and give nu-merical data for the existence of life in a water-dominant environment. Our conceptual experiment can be summarized by two steps. The first step of the experiment is to find hydrophobic organic materials floating inside the Europan oceans. This step aims to find a plausible form of life using one of the most fundamental features of life, the membrane. This step also can operate as a filter, which can prevent contamination from other samples (non-life, organic material-based objects). The second step is the analysis of the Europan organic materials to collect data that will be used to compare with the Earth biological database. Since the compositional data of Europa oceans is not available, the substances that would compose life on Europa are hardly predictable. To conquer this problem, matching the Europa data with terrestrial cellular profiles should be performed.
2 CONCEPTUAL DESIGN OF LIFE-DETECTING EXPERIMENT ON EUROPA
In this study, we designed an experiment with following assumptions:
-
1) Inside the thick icy shell of Europa, liquid-state water exists.
-
2) The designed experiment is conducted in laboratory conditions with samples from Europa.
Using metabolism to detect life is an incomplete experiment because of the diversity of life’s metabolism. Even on Earth, many species do not depend on glucose metabolism. So, the method used in 1976 is not considered universal.
On Earth, the major component of the cellular membrane is phospholipid, which is an amphipathic material (containing hydrophilic and hydrophobic affinities). Membranes of most of the life on Earth consist of a lipid bilayer, while some species consist of a lipid monolayer (Fig. 2). The reason why cells, the most fundamental element in life, choose to make their membrane with amphipathic material is because their free energy is lowest when amphipathic material forms a spherical structure. Water molecules are polar molecules, whose components possess partial electrical charges. In a waterdominant environment, charged molecules interact with water molecules by the process called hydration (Fig. 3). With respect to the oxygen molecule, which possesses a relatively negative charge, positive polar molecules tend to interact with the oxygen part of the water molecule. In terms of the hydrogen parts of the water molecule, the exact opposite mechanism happens. This process significantly lowers the free energy by the stabilization of each molecule through the electrostatic force.
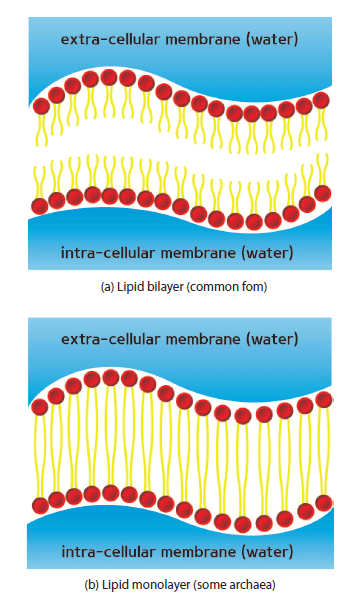
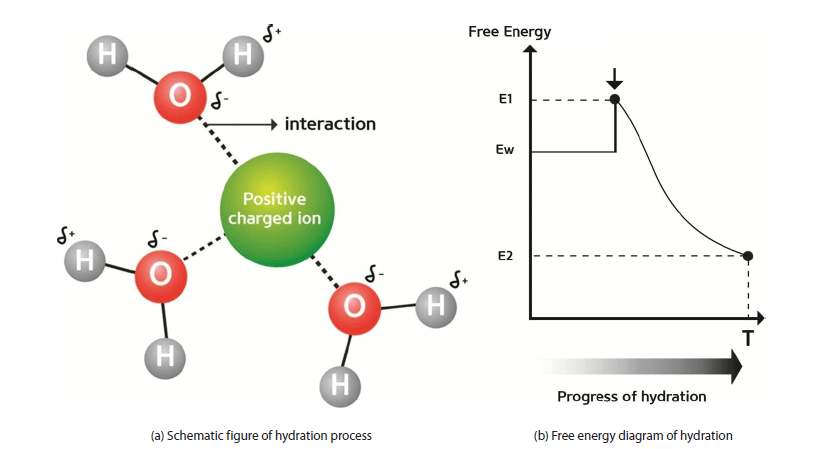
However hydrophobic materials do not interact with water molecules because they do not possess any electrostatic force. These molecules tend to interact with other hydrophobic molecules in a water-dominant environment through a process called hydrophobic interaction, which lowers their free energy (Fig. 4). Because each molecule holds others, the free energy of a system can be stabilized; however, since hydrophobic materials do not interact with water molecules, the total free energy of the system is slightly higher than that of a pure water system.
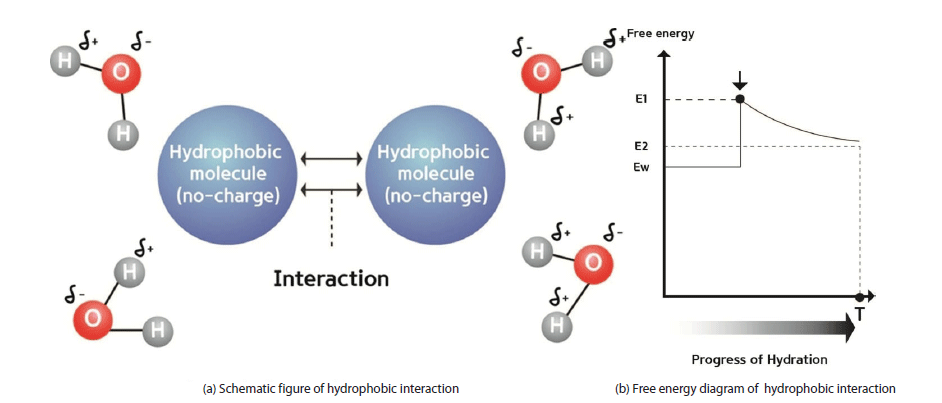
With these mechanisms, amphipathic molecules efficiently make stable structures, which can act as membranes. By placing the hydrophilic molecules in the radial and opposite directions, amphipathic molecules lock their hydrophobic molecules inside, causing these molecules to interact each other, which reduces the free energy of the system to its lowest possible state (Fig. 5). So, if life exists in Europa, we expect to find similar membrane structures that are made by amphipathic materials. Therefore, we suggest the search for lipid membrane-like structures as our first step of the experiment.
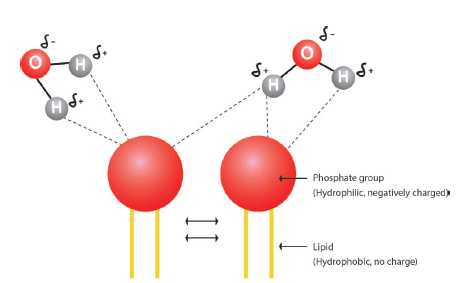
One considerable problem in using amphipathic materials as a proxy for life on Europa concerns the liposome. A liposome is an inanimate object that only consists of a lipid bilayer. The critical structural difference between a cell and a liposome is shown in Fig. 6. In the case of cells, a cellular membrane is filled with various functional groups to maintain a certain cellular environment (homeostasis). A liposome, however, is a spherical structure covered by amphipathic molecules. To conclude, the amphipathic structures are considered a proxy for life, a further step that can clearly show the life feature that is required.
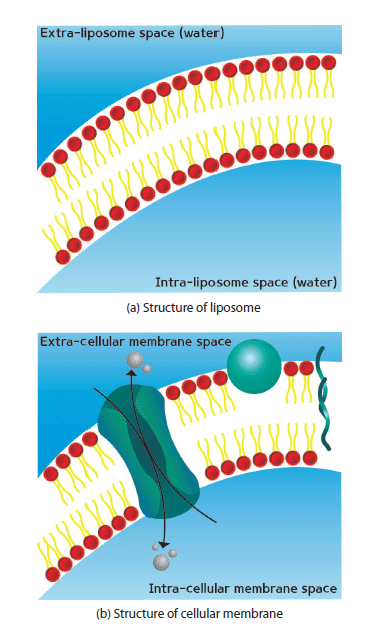
We suggest the next step of the experiment as comparing data of the membrane compositional constituents. Most of the functional groups of terrestrial life are is protein. However, there is no clear evidence that proteins are the universal constituent of a functional group of life. Searching for proteins in other worlds is not a suitable method to detect life, but comparing the compositional constituent data of amphipathic structures to Earth base life is expected to give an accurate probability of bio-signatures.. Also, it could serve as the astrobiological standard for searching alien biomaterials.
The chemical elements in the environment of the oceans of the Earth and Europa do not have to be identical. However, if alien life exists on Europa, the efficiency of metabolism should remain as high as possible to survive. Our experiment aims to detect unicellular life on Europa because multicellular organisms would not require a molecular-level detection experiment, considering the sizes of the samples on Earth. To maintain a metabolic high efficiency, on Earth, unicellular organisms have a size and chemical constituent ratio that remain in a relatively narrow range. Since an Europan alien cell membrane may have constituents that are not found on Earth, a method to infer the usage of each constituent should be developed. Our suggested method is to compare the membrane chemical profiles of unicellular organisms on Earth to Europa samples. This method is expected to guide the determination of the role of unknown substances.
To conduct the data comparison, Earth biological data should be systemized. For instance, the chemical constituents of the cellular membrane of various species should be input into our experiment database. Also, the data handling method should be developed to yield the probability of life from a targeted object. In this paper, we conducted a hypothetical experiment with only three hypothetical Europan samples. Used terrestrial data contains the most known samples (human erythrocyte, mouse liver cell, amoeba, data from Membrane Physiology, T.E. Andreoli 1978).
3 EXPECTED RESULTS
Fig. 7 shows a summary of the experiment. The detailed process and method to distinguish the spherical structures consisting of amphipathic molecules are not discussed in this paper. Fig. 8 shows the most abundant known cellular membrane chemical constituents (mass percentage). These chemical constituents -human erythrocyte, mouse liver cell and amoeba- are selected because their habitats are highly related to the existence of liquid-water. Distinguishing each substance can be done by mass spectroscopy. However, since the major components of Europan cellular membrane are unknown, they are presented as substances 1 through 4. Europa sample 1 is set as a possible life-form. Europa sample 2 is set as a liposome-like object, and Europa sample 3 is set as a vesicle containing an equal ratio of organic materials. Table 1 shows the numerical data of Fig. 8. Fig. 9 shows the data of hypothetical samples and its numerical data are shown in Table 2. Only the top four major constituents are considered in the figure of hypothetical data.
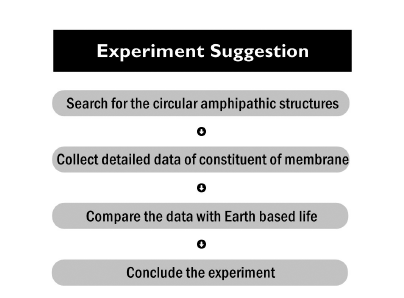
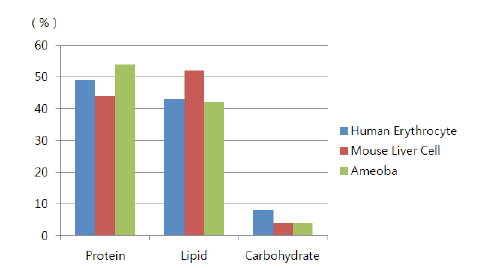
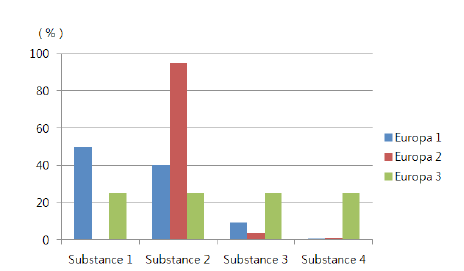
Protein | Lipid | Carbohydrate | |
---|---|---|---|
Human Erythrocyte | 49 % | 43 % | 8 % |
Mouse | 44 % | 52 % | 4 % |
Amoeba | 54 % | 42 % | 4 % |
Substance 1 | Substance 2 | Substance 3 | Substance 4 | |
---|---|---|---|---|
Europa 1 | 50 % | 40 % | 9.5 % | 0.5 % |
Europa 2 | 0 % | 95 % | 4 % | 1 % |
Europa 3 | 25 % | 25 % | 25 % | 25 % |
If the original features of the samples are unknown, because the samples were filtered once before the data analysis step, the first step of data analysis is to find the liposome-like structure. Liposome, shown in Fig. 8, mainly consists of amphipathic molecules. To assemble cellular organisms, liposome-like structures must have been formed before the emergence of the cell, especially in waterdominant environments, because they are the boundary line of life. So liposome-like structures are highly expected during the sample collecting step. From the numerical data, Europa sample 2 is highly expected to be a liposome-like structure because of a biased chemical constituent ratio (higher than 9:1). Also, the most abundant component of Europa sample 2 (substance 2) is the amphipathic molecule, which acts as phospholipid.
In the case of Europa sample 3, the chemical constituent of sample 3 does not match terrestrial life data. Also, inferring from the terrestrial data, the chemical constituent was mainly based on three major substances, lipid, carbohydrate, and protein. In contrast, Europa sample 3 consists of four equally distributed constituents, each of different substances. So judging from our limited data, Europa sample 3 is not classified as life. Europa sample 1 is concluded as life, matching well with terrestrial life data (human erythrocyte and amoeba). With this method and data, our conclusions are: Europa 1 as life, Europa 2 as liposome-like structure, and Europa 3 as non-life object. The integrated data of Europan and terrestrial samples is displayed on Fig. 10. With this method and data, our conclusion is: Europa 1 might be considered as life, Europa 2 as liposome-like structures and Europa 3 as non-life object.
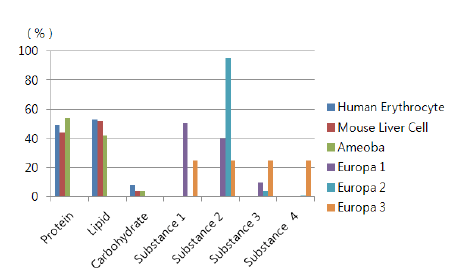
4 DISCUSSION AND CONCLUSION
In searching for life on other celestial bodies, performing an experiment depending on only one feature among the various features of life is not appropriate. Our suggested experiment is based on the morphological features of life, the most fundamental features of life with metabolism. If the existence of water in its liquid state on Europa is proven, our suggested experiment could give significant information as to the habitability of Europa. However, the accuracy of the experiment fully depends on the quantity and the quality of terrestrial life data. Membrane chemical constituent data of various species are limited. If the chemical constituent database of terrestrial live membranes is systemized, the analysis of our experiment could give broader information about the target.
Our experiment does not expect alien cells to consist of identical substances as the Earth. However, a similar constituent ratio is expected. The compositional ratio of terrestrial life membrane varies with species. For instance, the compositional ratio of lipids varies by 20 to 80 by mass, depending on the species. If a sufficient amount of data is not collected, our experiment could misguide the search for life on Europa. Detailed collection of data of cellular membranes, especially from microbes living in the deep oceans, is highly required.
Also, to analyze the data, if our experiment is sent to Europa, a program that can compare sample data to terrestrial data is highly required. Currently this program is being studied using “data mining” technology, which will give a more accurate probability of life. The program will distinguish life from inanimate objects using membrane constituent data. Further-more, it will give specific numerical probabilities through comparison with terrestrial data.