1 INTRODUCTION
Sea surface temperature (SST) is an important factor for understanding the climate system. SST analysis has been used to monitor and predict climate by converting irregular SST grid data to a regular grid (Smith & Reynolds 2003). The SST analysis data by the National Oceanic and Atmospheric Administration (NOAA) (Reynolds & Smith 1994; Reynolds et al. 2002) use infrared satellite data from the advanced very-high-resolution radiometer (AVHRR) and in situ data from ships and buoys on a 1° spatial grid from November 1981 to present. The new analysis SST data were developed using optimum interpolation (OI) at a spatial grid resolution of 0.25° (Reynolds et al. 2007).
El Niño is a large-scale oceanic warming phenomenon in the tropical Pacific Ocean that occurs on average every two to seven years. Many studies have explained this event as interactions between the tropical Pacific Ocean and the atmosphere. Bjerknes (1969) hypothesized a positive ocean– atmosphere feedback involving the Walker circulation. When the Pacific Ocean is under the normal condition, warm water accumulates west of the date line because the trade winds push water to the west. Air in the tropical western Pacific rises and then flows eastward. This is known as Walker circulation. The water flow is from east to west in the normal condition. During the El Niño period, a positive SST anomaly in the equatorial eastern Pacific decreases the east– west SST gradient and the strength of the Walker circulation (Gill 1980; Lindzen & Nigam 1987). The trade winds weaken, thus changing the ocean circulation and SST anomaly. This feedback produces the equatorial Pacific area warm condition (Philander 1981). The water flow is from west to east during the El Niño period (Bjerknes 1969; Philander 1990; McCreary Jr. & Anderson 1991; Neelin et al. 1998).
The Nino 1+2, Nino 3, Nino 3.4, and Nino 4 SST indices are used to monitor the tropical Pacific and are calculated based on SST anomalies averaged across a respective region (Rasmusson & Carpenter 1982). El Niño has been quantified by several indices as corresponding to times when SST anomalies exceed 0.5 °C in the Nino 3 region using a 5-month running average or 0.4 °C in the Nino 3.4 region using a 3-month running average (Trenberth 1997; Trenberth & David 2001).
2 DATA AND METHOD
Specifically, for the Pacific equatorial region, we used the NOAA OI SST V2 product (Reynolds et al. 2007), provided by the NOAA Earth System Research Laboratory (ESRL) Physical Sciences Division (http://www.esrl.noaa.gov/psd/data), to show the variation of the 2015 El Niño events. NOAA OI SST provides an alternate database of SST data that is a spatial grid resolution of 0.25° latitude × 0.25° longitude global grid (1,440 × 720) with a temporal resolution of 1 day.
We used a high-resolution OI of AVHRR infrared satellite SST data from a series of National Oceanic and Atmospheric Administration (NOAA) satellites. The NOAA Satellite, a series satellite, provides Earth's observation data to monitor global environment and understand our dynamic Earth. Table 1 show NOAA series satellites and AVHRR sensor type. The AVHRR is a radiation-detection imager that has four- or five-channel scanners and can be used remotely for determining cloud cover and surface conditions. They sense in the visible, thermal infrared, and infrared portions of the electromagnetic spectrum. The AVHRR dataset has been used in many fields. In the case of visible observation, the scanners have been used to monitor sea ice and snow or vegetation. The infrared observation can be used to measure sea surface temperature. Thus, the datasets from AVHRR can be used effectively to study global environmental change. We have summarized the characteristics and use of the AVHRR sensor (Table 2).
The SST data by AVHRR are available from late 1981 to 2016 and operational AVHRR data are provided for 2017 onward. There are two types of daily OISST (Reynolds et al. 2007). One product is the AVHRR-only product, which uses AVHRR data and in-situ data from ships and buoys. The other product (AVHRR+AMSR) uses AVHRR, advanced microwave scanning radiometer (AMSR), and in situ data.
In this paper, we selected the AVHRR-only dataset because this dataset includes consistent long-term collections over the world (Banzon et al. 2016). We averaged over thirty years (1982–2011) from the AVHRR-only dataset to obtain an average at 0.25° spatial grid points for one year. Then, we computed the SST anomaly from the selected base period (1982–2011). We identified the variation of SST during the 2015 El Niño period from the calculated SST anomaly data.
We also used the SST index data in the Nino 1+2, Nino 3, and Nino 4 regions to reveal warm water regions in the sea surface during the 2015 El Niño period. The Niño SST index data were calculated from the Hadley Centre sea ice and sea surface temperature data set (HadISST) from 1870 to Mar 2018 (Rayner et al. 2003). The Niño 1+2 index is the averaged SST anomalies within the region of 0–10°S and 90–80°W, the Niño 3 index is the averaged SST anomalies within 5°S–5°N and 150–90°W, and the Niño 4 index is the averaged SST anomalies within 5°S–5°N and 160°E–150°W.
3 RESULTS
We investigated the strong SST anomaly during the 2015 El Niño period to show the warm sea surface regions in the tropical sea surface area. Fig. 1 shows the sea surface temperature anomalies for June 2015 in the Niño 1+2, Niño 3, and Niño 4 regions when the El Niño warm phase began to spread around the equatorial region. The formation of El Niño is generally explained by a weakening or reversal from normal of the direction of the trade winds, which allows warm sea surface water to move eastward. In addition to the warm water coming from the west, we identified a warm water region in the Niño 1+2 and coastal Peru area, as shown in Fig. 1. We investigated the SST mean anomalies in the Niño 1+2, 3, and 4 regions to show the long-term variation of the sea surface temperature anomalies (Fig. 2). We found that the Niño 1+2 region has had a higher anomaly value than the Niño 3 or Niño 4 regions, as shown in Figs. 2 and 3. Fig. 4 shows a series of SST anomaly maps from January 2015 to June 2016. We used high-resolution satellite observations data for the SST maps, the NOAA OI SST V2 product (Reynolds et al. 2007), to show the warm water region of the El Niño event where water could be rising upward from below the Niño 1+2 region. The SST anomaly for the El Niño condition tends to peak in December, and the peak of the 2015 El Niño occurred in the winter of 2015–16 (Fig. 4). During the El Niño event, there was warm water in the Niño 1+2 and Peru coastal area from June 2015 to March 2016.
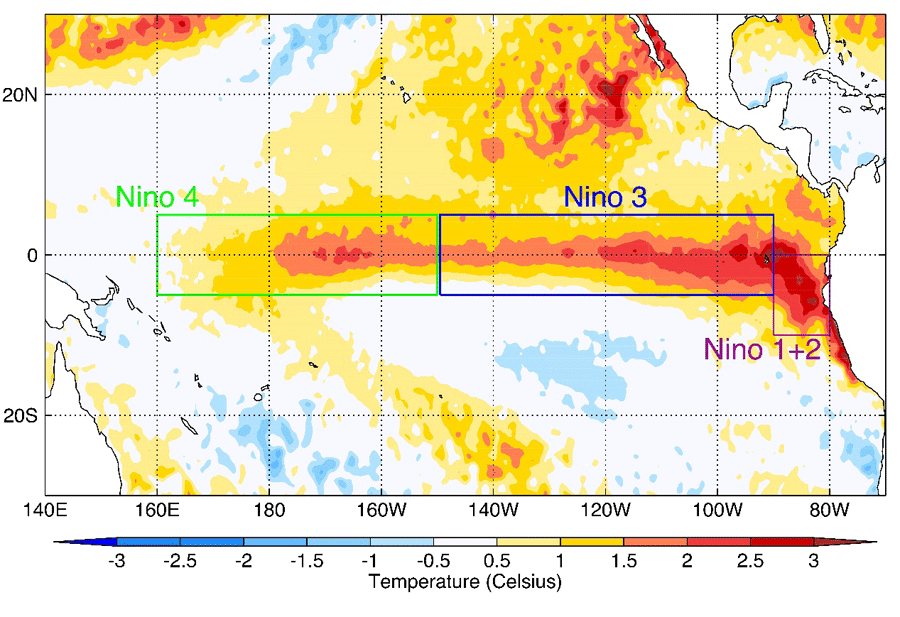
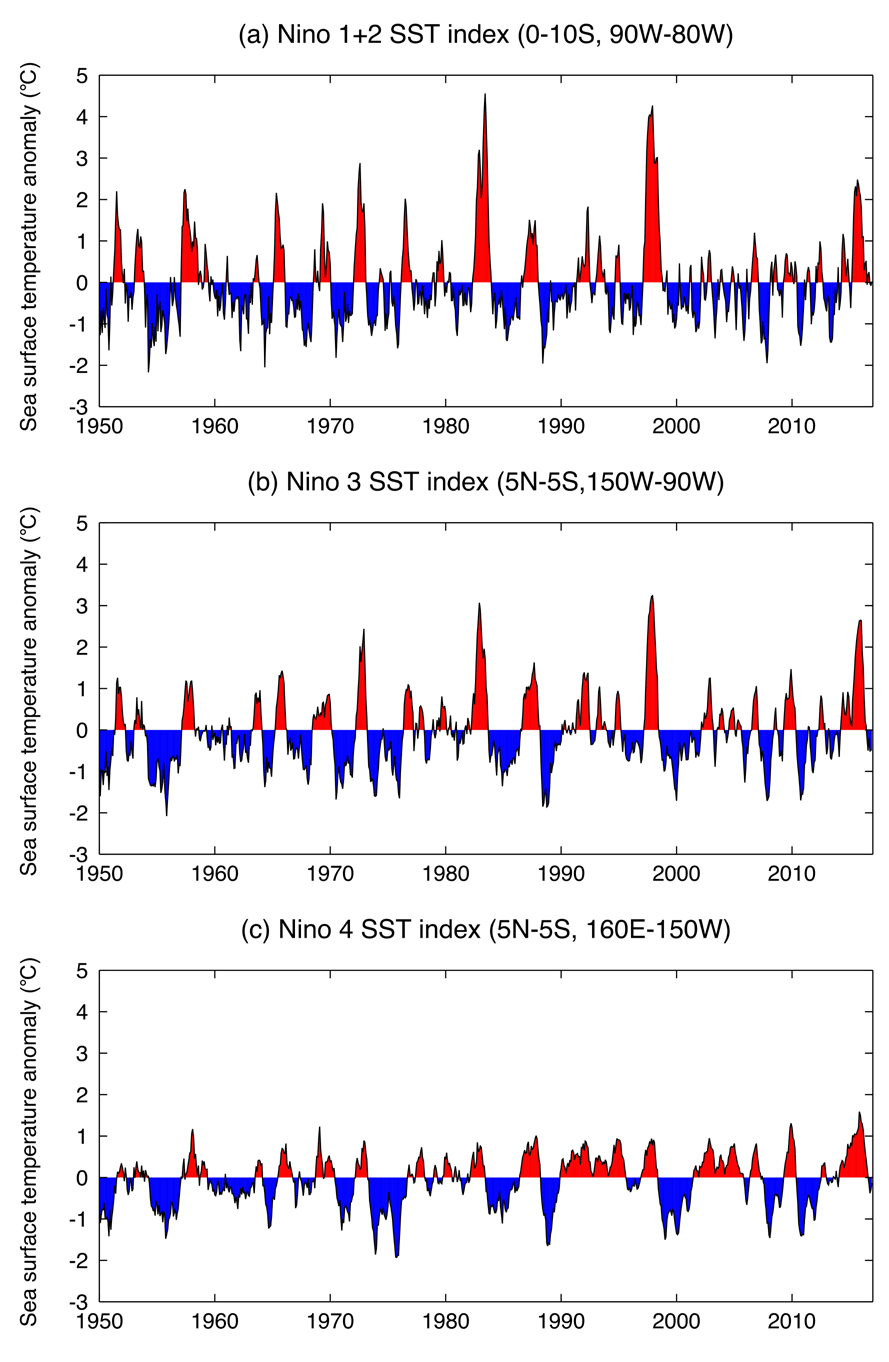
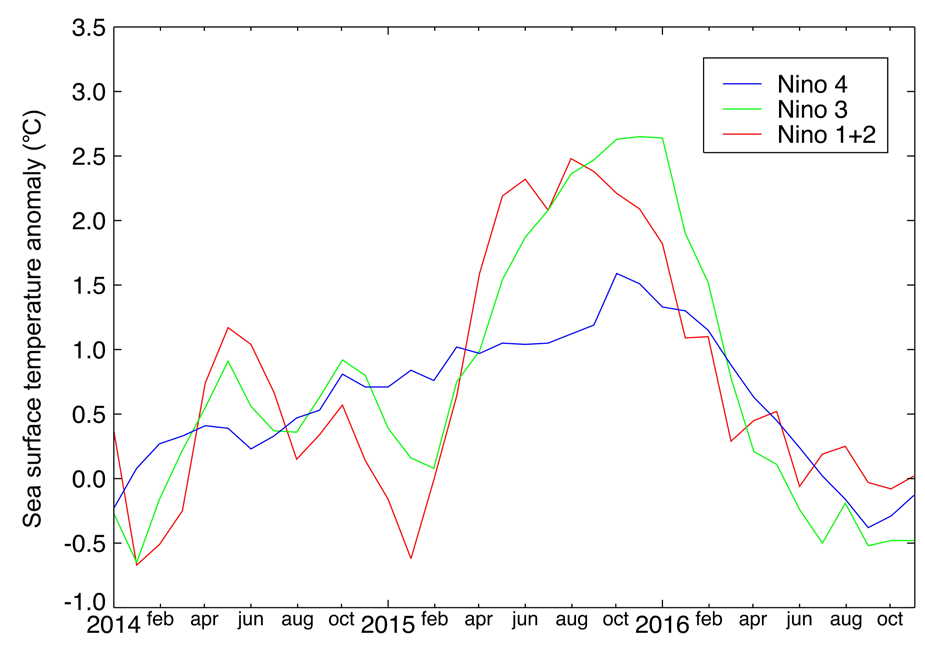
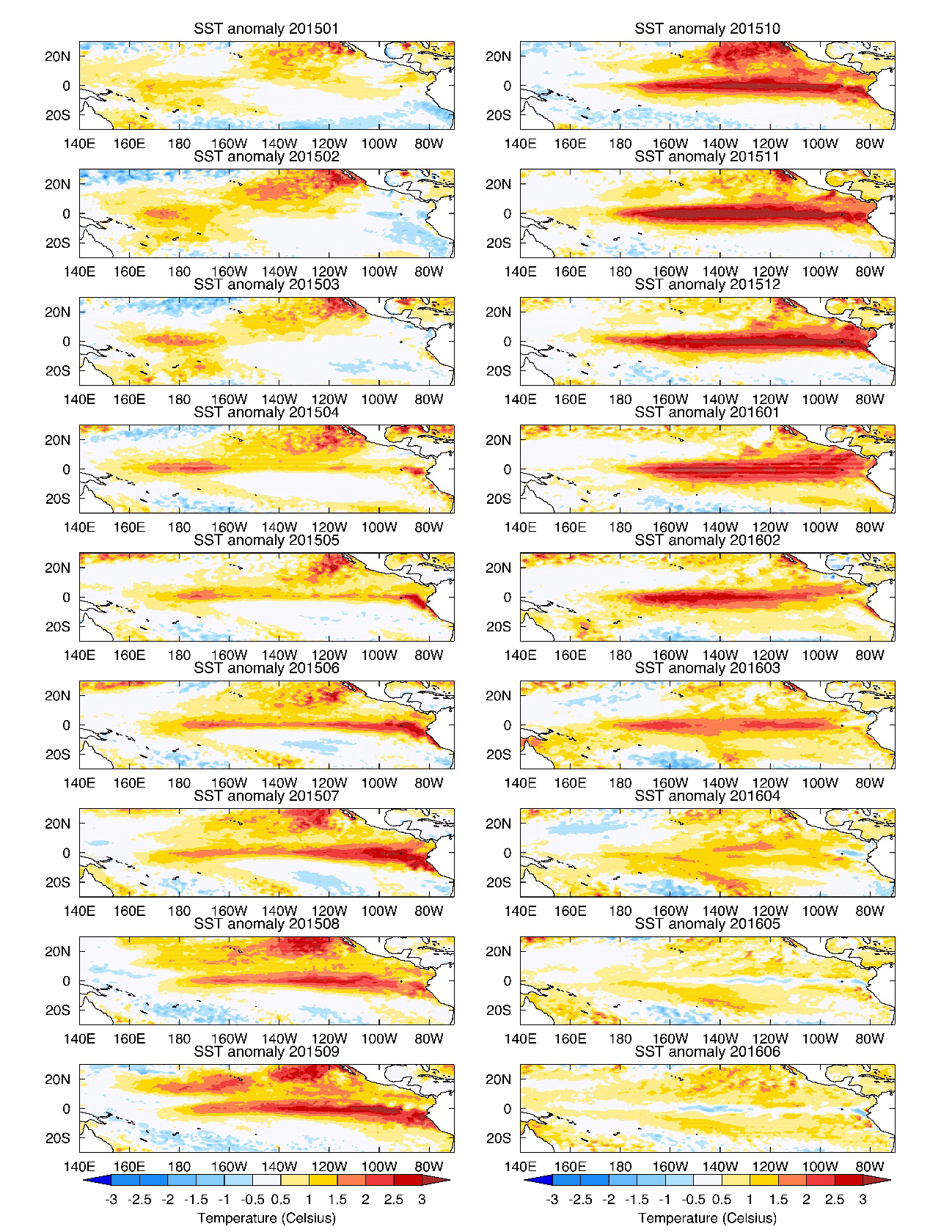
4 SUMMARY AND DISCUSSION
Normally, the movement of warm water in the western tropical Pacific Ocean is eastward during an El Niño period. We identified an additional warm water area in the Niño 1+2 region and the Peru coastal area using the high-resolution sea surface temperature dataset from the AVHRR and the Niño index supported by HadISST. The study of Lee & Yi (2016) suggested sources of heat from the bottom of the sea as a cause of melting of Arctic sea ice. We believe that the cause of this study are also applicable to the equatorial region. Therefore, to understand the mechanism of El Niño better, it is necessary to check both the surface condition and also the submarine condition.