1 INTRODUCTION
The Magellanic Clouds and our galaxy are members of the Local Group. Tidal interactions between the Large and Small Magellanic Clouds (LMC and SMC) and the Milky Way produce several high-velocity gas complexes connecting all three galaxies. The Magellanic Clouds appear to be optimal targets for investigations into stellar formation history in the Local Group. They have often been used to test the validity of galactic evolution theories. The high resolution spectroscopic observations of the brightest stars of these galaxies became available. As a rule, many spectroscopic analyses of the LMC/SMC supergiants only focused on the determination of elemental abundances for atomic numbers Z ≤ 64. Apparent progress achieved recently in the field of quantitative and experimental spectroscopy together with a continued improvement of the numerical codes used for the abundance analysis, made it possible to detect heavy elements in their stellar atmospheres such as, uranium and thorium.
These elements are produced in the universe as a result of neutron-capture processes; the so-called s- and r-processes of nucleosynthesis. Among other factors, the yield of these neutron-capture elements may depend upon the overall metallicity of the progenitor star. It is well known that the metal content of the Magellanic Clouds is lower than that of our galaxy. This leads to an investigation on specific conditions of their chemical evolution that is reflected in the peculiar elemental distribution observed in their stellar population.
Note that the final evolutionary stage of red supergiants was usually discussed as a supernova outburst. Recently (Kochanek et al. 2008; Adams et al. 2017a, b), it was found that a significant fraction of red supergiants, namely 0.04- 0.47 (with the most reliable fraction near 0.14) can disappear without explosion; the core collapse of a massive star decreases the brightness of an initial source below the detection limit. This observational result shows the necessity of investigations into the individual supernova progenitors to separate criteria for outbursts, or shows that the core collapse evolutionary path is not yet clearly understood.
In this paper, we derive the elemental abundances in LMC supergiant star RM_1-390, with an emphasis on a detailed investigation into the s- and r-process elemental abundances. We determined the abundances of 19 elements in the atmosphere of RM_1-390 and compared its abundance pattern to other LMC and SMC supergiants.
2 SPECTROSCOPIC OBSERVATIONS AND ATMOSPHERIC PARAMETERS
The high resolution spectral observation of RM_1-390 was obtained from the 3.6 m telescope at the European Southern Observatory by Hill (1997). The spectrum was obtained by V. Hill, but was not used in her publications. This paper is the first to determine the abundance pattern for RM_1-390. The signal-to-noise ratio (S/N) of the spectrum is greater than 100 (S/N > 100) while the resolving power was equal to R = 20,000 and the wavelength coverage is from 5,850 Å to 7,095 Å with several gaps between orders. The extraction of the observed spectrum from charge-coupled device (CCD) imaging and its wavelength calibration were made by Hill (1997). The next reduction steps are the determination of the continuum level and then the identification of spectral lines made with URAN (Yushchenko 1998) and SYNTHE (Kurucz 1993) codes. Fig. 1 shows an example of the observed spectrum.
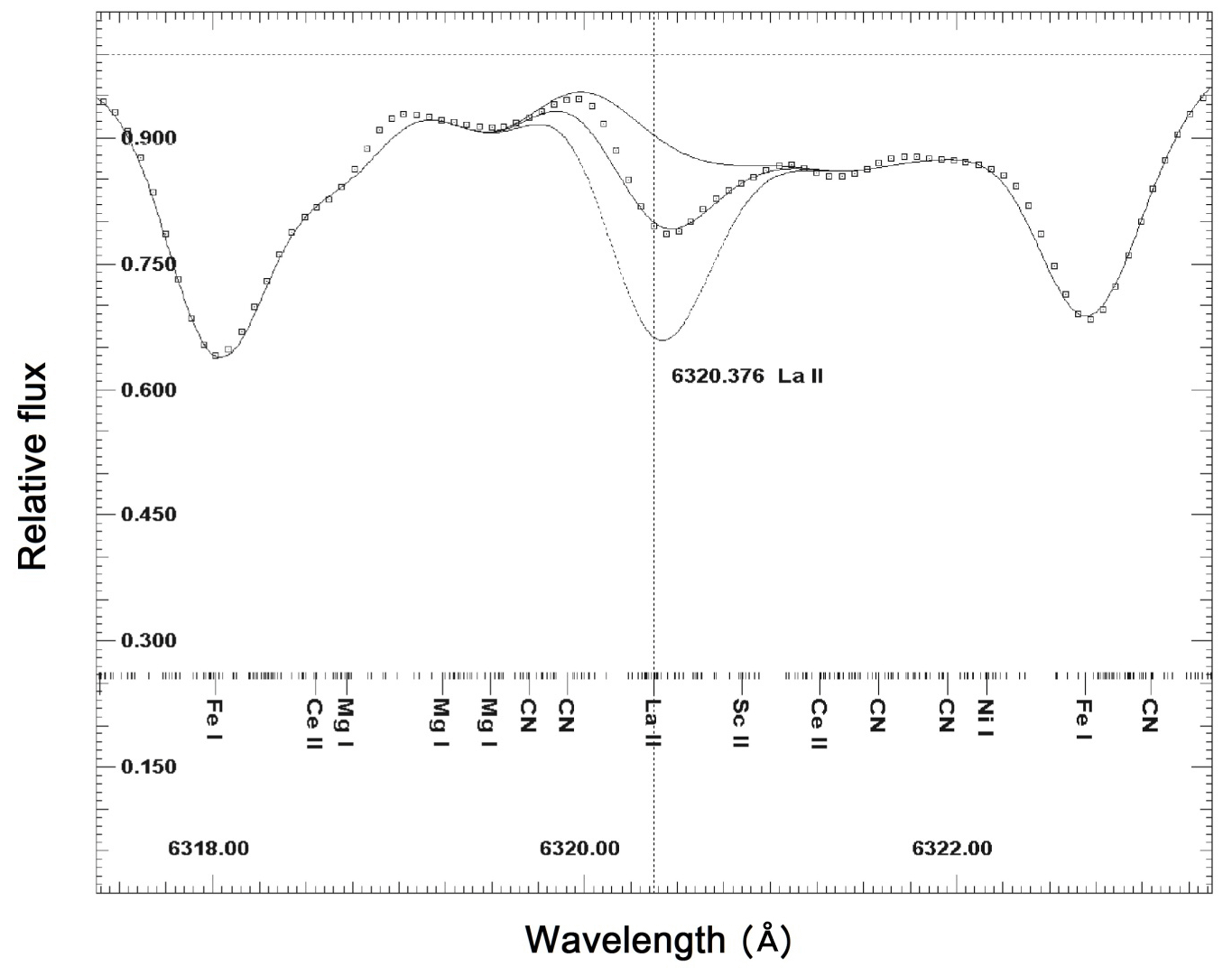
The list of atomic and molecular lines was taken from Kurucz (1993), Hirata & Horaguchi (1995), Morton (2000), DREAM data base (Biémont et al. 2017), VALD data base (Piskunov et al. 1995), Fuhr & Wises (2006) and other sources. The clean and not strongly blended lines in the observed spectrum were selected on the basis of comparison with the synthetic spectrum. The detailed calculation procedure can be found in Yushchenko et al. (2015) and Kang et al. (2013).
The effective temperature, surface gravity and other stellar parameters were found using the method, developed by Yushchenko et al. (1999) and Gopka et al. (2004). This method uses the iron abundances calculated from various spectral lines under the different stellar atmosphere models to determine the stellar parameters. The grids of the atmosphere models with effective temperatures from 3,900 K to 4,500 K (step 10 K) and surface gravities from log(g)=0.00 to 0.50 (step 0.02) were prepared by the interpolations of Kurucz (1993) atmosphere models. Afterwards, the following parameters were derived: the effective temperature Teff = 4,250 ± 50, the surface gravity log g = 0.16 ± 0.1, the microturbulent velocity vmicro = 2.5 ± 0.1 km/s, and the iron abundances [Fe/ H] = -0.73 ± 0.11. Thirty-nine lines of neutral iron and 10 lines of ionized iron were used for these calculations. The errors pointed out are estimated from the errors of the used method. The level of the systematic errors can be higher, as illustrated in Table 1.
Table 1 shows the spectral types, colors and radial velocities of the Magellanic Clouds red supergiants observed by Hill (1997). Their temperatures are taken from Massey & Olsen (2003) for RM_1-390, from Gopka et al. (2012a) for RM_1-667, and from Hill (1997) for the rest of the stars. The references for their spectral types and colors are included in the table. Their published radial velocities, and the minimum and maximum values with the differences between these extrema are taken from the SIMBAD data base.
The temperature of RM_1-390 was found to be 3,200 K by Massey & Olsen (2003). This value is a thousand degrees lower than our determination and needs to be discussed. It is to be noted that B-V color for PMMR144 and U-B colors for RM_1-390 and RM_1-667 are not usual for late type stars. One of the possible explanations for these can be the existence of a close blue companion; a member of binary system or a background/foreground star. In the case of a binary system, the variations of radial velocities should be observed. Two of the aforementioned three stars, namely PMMR144 and RM_1-390, exhibit the greatest differences between the minimum and maximum published radial velocities, 38.9 and 12.5 km/s, respectively. We can conclude that the low effective temperature value for RM_1-390, published by Massey & Olsen (2003), can be explained by an unresolved binary companion. The case of real physical variations is also possible; Szczygieł et al. (2010), found the brightness varies between V = 12.2 to 12.7 at the time scale near ten years and nearly one year with an amplitude of almost 0.1 magnitude. Our set of parameters found for the moment of the spectral observation was used to derive the chemical composition of RM_1-390.
The abundances of all chemical elements except for iron were found using the spectrum synthesis method. It requires additional information to take into account the broadening effect of the spectral lines. It was assumed that the broadening is due to the macroturbulence in the atmosphere of RM_1-390. The value of the macroturbulence velocity was found by fitting the profiles of clean lines of neutral iron to the synthetic profiles, calculated with different values of the macroturbulence velocity. The best fit was found for the value, vmacro = 9 km/s.
3 CHEMICAL COMPOSITION
To derive the elemental abundance in our program stars, we used the synthetic spectrum technique. The synthetic spectra were generated using Kurucz’s (1993) code, SYNTHE. The fit of the observed spectrum with the synthetic one was made with the URAN code (Yushchenko 1998). Hyperfine and isotopic splitting are taken into account for some elements such as Mn, Co, Ba, and Eu, using the data (mainly) from Kurucz (1993). To avoid some possible errors in the oscillator strengths, we tried to find the counterparts of investigated absorption lines in the solar spectrum. The Liege Solar Atlas (Delbouille et al. 1973) and the Grevesse & Sauval (1999) solar photosphere model were used to find the abundances in the solar atmosphere. The continuum in the Liege Solar atlas was corrected in accordance with the works of Ardeberg & Virdefors (1979) and Rutten & van der Zalm (1984). A more detailed description of the method can be found in Yushchenko et al. (2015), and Kang et al. (2013).
Based on the LTE approximation, both the plane-parallel (Kurucz 1993) and the spherically symmetric (Gustafsson et al. 2003) atmosphere models were used. The fit of the observed spectrum by the synthetic one in the vicinity of lanthanum line is shown in Fig. 1.
Table 2 summarizes the abundance calculations. The first three columns of the table are the atomic number, the designation of each chemical element with its ionization and the number of used spectral lines. In columns four through seven, one can find the mean chemical abundances in the atmosphere of RM_1-390 with respect to the solar photosphere abundances. The last figures in parenthesis after the corresponding relative abundances show the calculated errors. Four atmosphere models were used, one model for the stellar parameters, described in the previous section, another one for the surface gravity increased by 0.2 dex, another one for the effective temperature increased by 100 K and the last model from the MARCS grid (Gustafsson et al. 2003).
The abundances of 18 chemical elements from silicon to thorium were found in the atmosphere of RM_1-390 from the model synthesis. Fig. 2 shows the abundance patterns of RM_1-390.
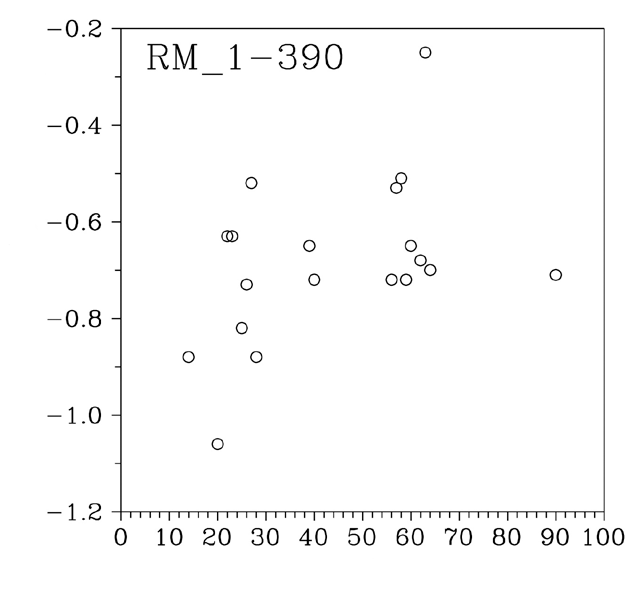
4 DISCUSSION
All chemical elements in the atmosphere of RM_1-390 show the underabundances with respect to the solar values. The values of underabundances are close to -0.7 dex; the iron deficiency in the atmosphere of this star. It is not a surprising result given that the deficiency of chemical elements in the Large Magellanic Cloud is a well-known fact, the mean metallicity of LMC is [Fe/H] = -0.37 ± 0.12 (Choudhury et al. 2016). The distribution of individual chemical elements in the abundance patterns of the various LMC stars is more interesting. Fig. 2 shows that the lowest relative abundance is observed for calcium while the highest is observed for europium. The europium and other neutron capture elements will be discussed next.
Fig. 3 compares the abundances of the r- and s-process elements in the atmosphere of RM_1-390 with the solar system distributions of these elements. This allows us to claim that the r-process elements in the atmosphere of RM_1-390 exist, but the role of the s-process is more important than in other Magellanic Clouds red supergiants. For example, we can point out the investigation by Gopka et al. (2013b), where the abundance patterns of PMMR23, PMMR144, and RM_1-667 were found, and also another by Gopka et al. (2012b, 2013a) where the absorption lines of osmium and thorium were found in the spectra of several other red supergiants; PMMR23, PMMR144, and RM_1-667 exhibit the excess of the r-process elements.
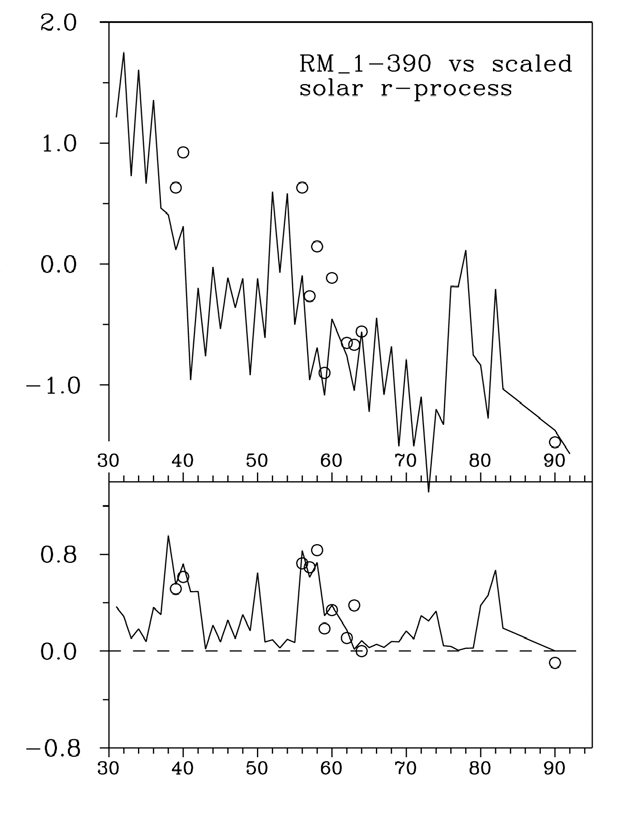
RM_1-390 is the first star in our sample without the overabundances of the r-process elements with respect to iron. It should be noted that the r-process elements lines can be detected. As an example of this, we can point to two thorium lines in the spectrum of RM_1-390, identified by Gopka et al. (2013a), and europium and gadolinium lines in this study, but the deficiencies of thorium and gadolinium are close to that of iron.
It is necessary to note that RM_1-390 is one of the strongest infrared objects in the LMC. It is detected at a wavelength as long as 24 microns (Bonanos et al. 2009) and the significant infrared excess is observed at wavelengths longer than 8 microns. That is why it is possible to expect the existence of the circumstellar envelope. To detect the possible signs of this envelope, we plot the dependencies of the relative surface abundances in the atmosphere of RM_1-390 as a function of the second ionization potentials (Fig. 4) of the corresponding chemical elements.
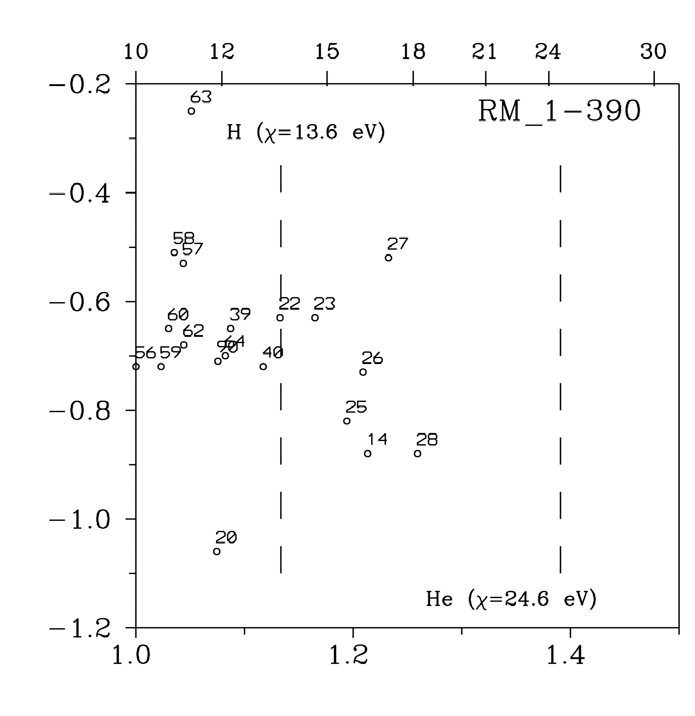
The most interesting feature of Fig. 4 is the deficiency of calcium. It was first pointed by Greenstein (1949) and can be explained by the charge-exchange reactions of calcium and hydrogen atoms. Unfortunately, this hypothesis is not fully developed yet. Recently, Yushchenko et al. (2015) made a review devoted to this problem, and found some new possible observational confirmations. One of them is the correlation between relative surface abundances and the second ionization potentials in the range from 12.5 to 20 eV for the groups of stars with different effective temperatures. As shown by Yushchenko et al. (2015, Fig. 7), this correlation is positive for the groups of stars with effective temperatures in the range of 7,000-12,000 K, and negative for groups of stars with lower temperatures, specifically in the 5,900-7,000 K range. Maybe it can be explained by the transition from radiative to convective atmospheres. Our preliminary results show a positive correlation for red giant stars, where the radiative energy transfer becomes significant again.
We found the abundances of eight chemical elements with their second ionization potentials from 12.5 to 20 eV in the atmosphere of RM_1-390. The correlation coefficient between the abundances and the second ionization potential is negative, specifically ρ = -0.33 ± 0.32. The value of the error is defined mainly from the case of cobalt, which has the highest relative abundance in this range of potentials. It should be noted that the abundance of cobalt was determined by only one line. The relative abundance of cobalt in the surveys used by Yushchenko et al. (2015) was also higher than the relative abundances of other chemical elements in this range of the second ionization potentials. Without cobalt, the correlation coefficient is ρ = -0.77 ± 0.15. If the Greenstein (1949) hypothesis can explain the values of the correlation coefficients found by Yushchenko et al. (2015), it is possible to conclude that RM_1-390 has some significant convective energy transfer in its atmosphere.
It is to be noted that the Greenstein (1949) hypothesis was mentioned to explain the peculiar abundances in binary stars (Kang et al. 2012, 2013; Jeong et al. 2017). It was shown that the correlations between relative abundances and second ionization potentials of corresponding elements were observed not only in radiative atmospheres, but also in convective atmospheres in the case of strong flux accreted from the circumstellar envelope.
It is also necessary to mention a possible detection of Thorne-Żytkow objects (Thorne & Żytkow 1975). These objects have a massive nondegenerate envelope surrounding a degenerate neutron core. It can be the result of a strong accretion process onto an existing neutron star. These objects should have effective temperatures similar to those of red supergiants and their radii near 103 solar value. The observational properties of Thorne-Żytkow objects are very close to the properties of red supergiants, as such it is very difficult to identify such objects. Only few candidates have been discovered thus far (Levesque et al. 2014). ThorneŻytkow objects should show strong lithium and some heavy elements lines in their spectra. The abundance pattern of RM_1-390 rejects this possibility as the relative abundances of yttrium and zirconium are of the same order as that of iron.
We can conclude that RM_1-390 has unusual colors, strong infrared excess and, possibly, variable radial velocities. The absorption lines of the both s- and r-process elements are detected in its spectrum. The abundance pattern can be fitted to the solar system s-process curve. The atmosphere of RM_1- 390 shows signs of accretion of interstellar gas. The signs of some important convective energy transfer are detected in the atmosphere of this supergiant. All these features are consistent with the possible existence of a binary satellite and the strong circumstellar envelope.