1 INTRODUCTION
Aviation space weather is a very active area of research because air safety is tied to the phenomenon of ionizing radiation from space. There are three main origins of space radiation residing within the Earth’s atmosphere; these include galactic cosmic rays (GCRs) from deep space, solar energetic particles (SEPs) from the Sun, and trapped energetic protons/electrons in the Earth’s radiation belts. Space radiation consists of ionizing radiation that ionizes the incident energetic particles in the atmosphere via interactions with atmospheric neutral atoms, and this produces secondary radiation particles. Generally, relatively low energy particles are cutoff by the Earth’s geomagnetic field, but relatively higher energy particles (i.e., those higher than a few tens of mega electron volts) can penetrate into the atmosphere and cause harmful aviation radiation. Korean airline companies commonly operate along polar routes where radiation levels can be high, and these companies as well as space weather service providers are paying greater attention to the monitoring and prediction of aviation radiation doses. In fact, various efforts have been conducted in Korea for that purpose; as part of these efforts, in-flight measurement experiments of space radiation were performed in 2009 to ensure the safety of flight crews and passengers over polar routes (Hwang et al. 2010). Furthermore, the development of a new aviation radiation estimation model based on GCRs and SEPs was also carried out (Hwang & Shin 2013; Hwang et al. 2014). A global framework for addressing radiation issues in regard to the space weather environment has been recently been constructed, but more must be done at the international and national levels (Tobiska et al. 2015).
The CARI-6/6M programs are currently the most widely used programs globally for the estimation of the aviation radiation dose, and these programs can be obtained from the U.S. Federal Aviation Administration (FAA) via a homepage (https:// www.faa.gov/data_research/research/med_humanfacs/ aeromedical/radiobiology/cari6/download/) (Copeland 2013) as a simple execution file. The most important input parameter for the CARI-6/6M programs is the heliocentric potential (HCP) value. The HCP value is derived from ground neutron monitoring observations, but the exact calculation method is not well known. Though almost all aviation companies in the world use this HCP value as displayed on the FAA webpage to run the CARI-6/6M programs, this critical value is not provided promptly, i.e., the data are actually provided at delay time of one or two months. This makes difficulties for aviation space weather customers to nowcast or predicts the aviation route dose. So for the purpose of helping space weather operators solve this practical problem, we recently developed a HCP prediction model based on monthly sunspot numbers (Hwang et al. 2015). By using this HCP prediction model, we can predict the HCP value up to eight months in advance. In this study, we tested the accuracy of our HCP prediction algorithm and worked on improving the model. Specifically, we tried various intermediate processes to extract the HCP value from the sunspot number, which involved using the monthly sunspot, daily sunspot, and real neutron count observations collected at the Apatity Cosmic Ray Neutron Count Monitor.
2 HCP PREDICTIONS FROM THE SUNSPOT NUMBER
Based on the relationship between the monthly sunspot number and the monthly HCP value, we found the following functional form for the HCP value (Hwang et al. 2015); hereafter, this is referred to as the HCP prediction model:
Here, S is the average monthly sunspot number eight months prior to the HCP(MV). In contrast, the FAA provides monthly HCP values at a delay time of one or two months; the method using this delayed HCP is indicated as the “Standard” type in Table 1. We also considered three other methods to obtain the HCP value directly from the observed sunspot number data, and these are indicated as type A, B, and C in Table 1. To decide the most accurate method to obtain the HCP value, we implemented the three approaches as follows. Type A involved obtaining the monthly HCP by using the HCP prediction algorithm with the monthly averaged sunspot data, and the monthly aviation route dose was calculated by using the CARI-6M program. Type B employed the daily predicted HCP calculated from the daily sunspot number, and those daily HCP values were averaged for one month to calculate the monthly aviation route dose. Type C also used the daily sunspot number and daily HCP values, but a daily effective dose was computed, and these numbers were averaged on a monthly basis. We used the HCP values from January 2014 to February 2016 in the analysis, and the sunspot number data were from May 2003 to June 2015.
Fig. 1 shows the plot obtained by applying the methods described in Table 1 to a specific location (latitude 79° N, longitude 169° E) along the polar route at an altitude of 10 km. There were no large differences among the results for the type A, B, and C approaches. To quantify the differences between the standard method and types A, B, and C, we calculated the root mean square error (RMSE) according to different altitudes. These results are shown in Table 2. We found that the RMSE increased as the altitude increased, and the most consistent result with the standard method was obtained with type C, which was associated with the smallest RMSEs for all the altitudes.
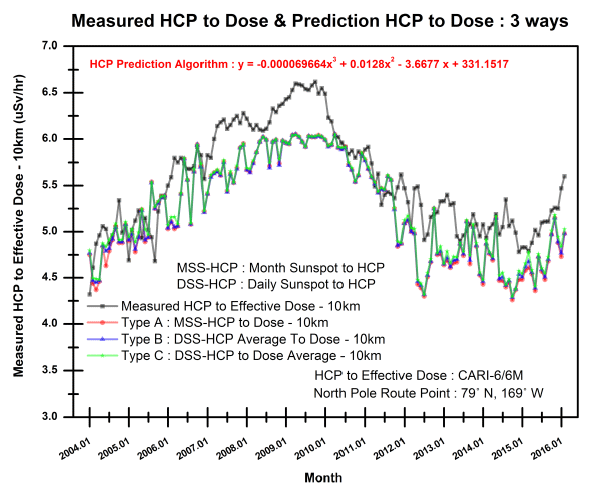
RMSE Analysis | 0 km | 5 km | 10 km | 15 km |
---|---|---|---|---|
standard & type A | 0.001109 | 0.037235 | 0.430564 | 1.361126 |
standard & type B | 0.001083 | 0.036526 | 0.422074 | 1.338522 |
standard & type C | 0.001055 | 0.035041 | 0.404729 | 1.278379 |
3 HCP PREDICTIONS BY USING THE NEUTRON MONITORING OBSERVATIONS
As shown in Fig. 2 and Table 2, the HCP prediction algorithm was suitable except for a few singularities near the maxima and minima. Additionally, the residual was the highest near the solar maximum and the solar minimum; this occurred because cosmic rays are the most intense near the solar maximum, and solar proton events occur most frequently near the solar maximum. The differences between two effective doses derived by the standard method and type C approach ranged from -1.0 to 0.5 μSv/hr, and the maximum of the mean absolute percentage error (MAPE) was about 6.5 % (Fig. 3).
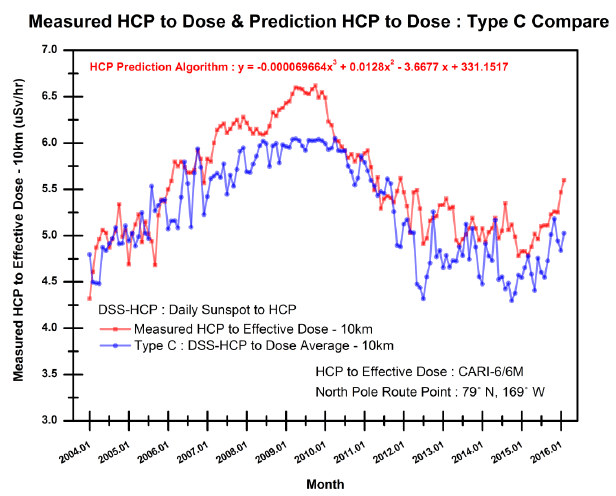
To obtain more accurate HCP values and effective doses, we considered using the neutron counts directly. Specifically, we used the Apatity neutron monitoring data. The Apatity cosmic ray station is located at 67.57° N, 33.39° E, and at an altitude of 181 m above sea level. The effective vertical cutoff rigidity at the station’s geographic location is 0.65 GV. The detector is a standard 18-NM-64 neutron monitor type, and the station has provided continuous 1 hr neutron data since May 1969. Fig. 4 shows the relationship between the neutron counts observed at the Apatity ground station and the HCP values. The HCP values were anti-correlated with the neutron counts as expected. Furthermore, the HCP values were anti- correlated with the effective dose rates. We also can infer then that the neutron count observations should be correlated with the effective dose, and Fig. 5 shows this relationship. In this figure, the neutron counts changed consistently with the effective dose rate except for the very recent outlier in 2016, which might have been due to the fact that provisional HCP values were used for this date in 2016.
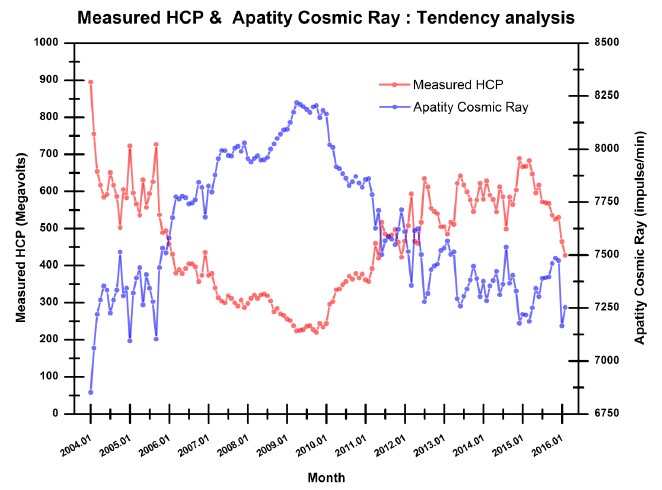
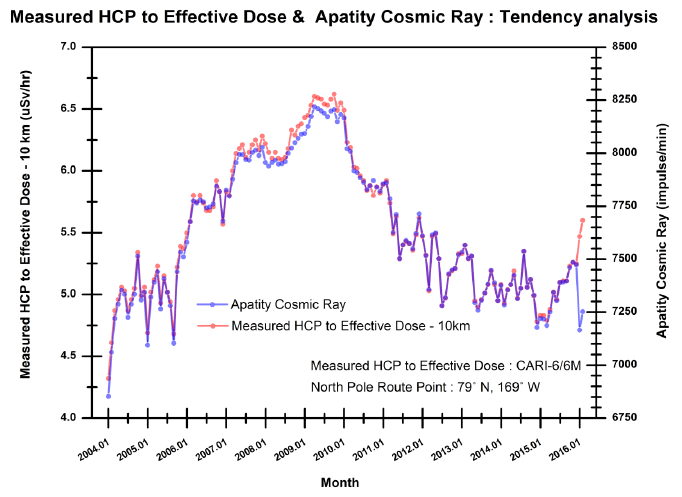
Fig. 6 shows a scatter plot between the HCP values provided by the FAA and the cosmic ray observations at the Apatity ground station; the data displayed a clear anti-correlation. The fitting function between the two parameters is as follows:2
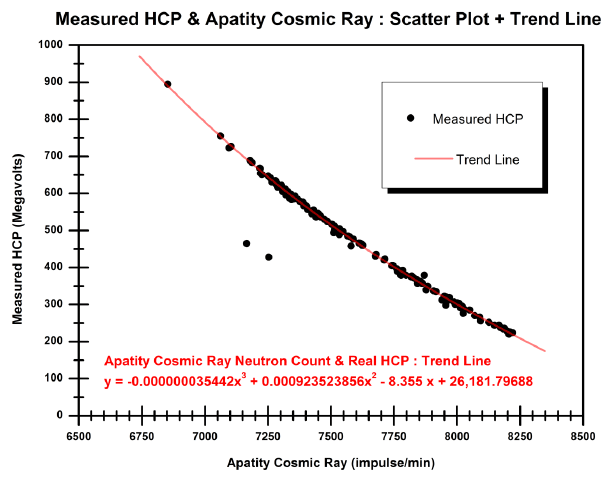
Here, x indicates the observed neutron counts at the Apatity station. Now, with this formula, we can obtain the real-time HCP values directly derived from the ground neutron monitoring observations. Thus, this can fill in the gap of one or two months of HCP delay caused by late delivery from the FAA. Fig. 7 shows the comparison between the HCP values provided by the FAA and the HCP values derived from the neutron counts. These two parameters showed very similar trends except for a recent few points in 2016. For these exceptional cases, we do not know the exact reason for the discrepancy; it might have been caused by the provisional HCP values used or unexpected changes in the HCP calculation algorithms inside CARI-6M by the FAA. We will leave this topic for future work. Fig. 8 shows the differences between two HCP values, which were either obtained from the FAA webpage or derived from the cosmic ray association indicated by red and blue lines in Fig. 7. The maximum difference was ~25 MV (2.7 % of the maximum HCP value). Generally, the differences were quite small and can be considered negligible.
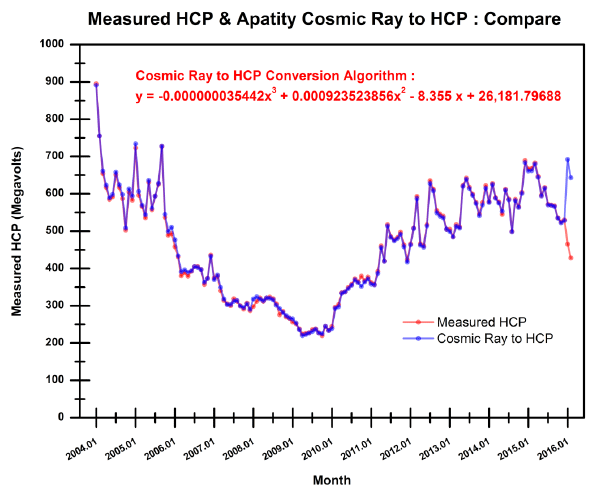
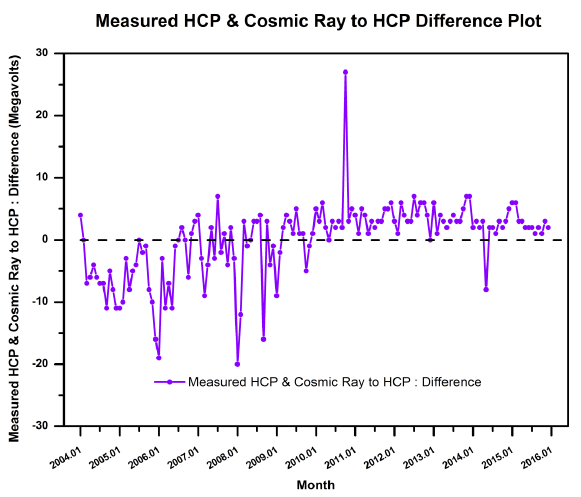
4 CONCLUSIONS
We developed the HCP prediction algorithm based on the observed sunspot number to overcome the limitation of CARI-6/6M programs for aviation space weather customers (Hwang et al. 2015). Originally, our HCP prediction model produced monthly HCP values because the HCP values were put on the FAA webpage as just a monthly HCP value, which was used as an input value for CARI-6/6M. However, in the real world, space weather customers need daily and real-time HCP values. Actually, they require daily effective doses over entire air routes to assist the aviation companies in protecting the aircrews, pilots, and public from harmful radiation. Hence, we investigated predicted daily HCP values and resultant daily effective dose rates by employing a different approach. Finally, we found that the type C approach, which uses real-time daily sunspot data to predict daily HCP values and calculate daily effective doses, was the most accurate of the three alternative approaches that were tested.
We also developed a calculation algorithm for the HCP values that uses direct neutron counts observed at the Apatity ground cosmic ray station. This simple formula is similar to the HCP prediction algorithms we developed for using the sunspot number (Hwang et al. 2015). From this newly developed formula, we can obtain the real-time HCP values from the neutron count data directly. This can thus contribute to filling in the gap in HCP values caused by the delayed delivery of FAA HCP data on the official webpage.